Surface geochemistry of the clay minerals
- PMID: 10097044
- PMCID: PMC34275
- DOI: 10.1073/pnas.96.7.3358
Surface geochemistry of the clay minerals
Abstract
Clay minerals are layer type aluminosilicates that figure in terrestrial biogeochemical cycles, in the buffering capacity of the oceans, and in the containment of toxic waste materials. They are also used as lubricants in petroleum extraction and as industrial catalysts for the synthesis of many organic compounds. These applications derive fundamentally from the colloidal size and permanent structural charge of clay mineral particles, which endow them with significant surface reactivity. Unraveling the surface geochemistry of hydrated clay minerals is an abiding, if difficult, topic in earth sciences research. Recent experimental and computational studies that take advantage of new methodologies and basic insights derived from the study of concentrated ionic solutions have begun to clarify the structure of electrical double layers formed on hydrated clay mineral surfaces, particularly those in the interlayer region of swelling 2:1 layer type clay minerals. One emerging trend is that the coordination of interlayer cations with water molecules and clay mineral surface oxygens is governed largely by cation size and charge, similarly to a concentrated ionic solution, but the location of structural charge within a clay layer and the existence of hydrophobic patches on its surface provide important modulations. The larger the interlayer cation, the greater the influence of clay mineral structure and hydrophobicity on the configurations of adsorbed water molecules. This picture extends readily to hydrophobic molecules adsorbed within an interlayer region, with important implications for clay-hydrocarbon interactions and the design of catalysts for organic synthesis.
Figures
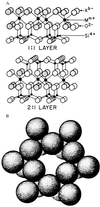
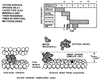
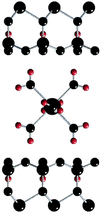
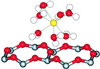
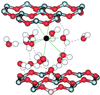
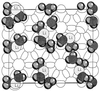
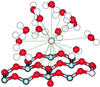
Similar articles
-
Atomistic simulations of cation hydration in sodium and calcium montmorillonite nanopores.J Chem Phys. 2017 Aug 28;147(8):084705. doi: 10.1063/1.4992001. J Chem Phys. 2017. PMID: 28863548
-
Formation of replicating saponite from a gel in the presence of oxalate: implications for the formation of clay minerals in carbonaceous chondrites and the origin of life.Astrobiology. 2012 Jun;12(6):549-61. doi: 10.1089/ast.2011.0635. Astrobiology. 2012. PMID: 22794298
-
Effects of Layer-Charge Distribution of 2:1 Clay Minerals on Methane Hydrate Formation: A Molecular Dynamics Simulation Study.Langmuir. 2020 Apr 7;36(13):3323-3335. doi: 10.1021/acs.langmuir.0c00183. Epub 2020 Mar 16. Langmuir. 2020. PMID: 32109063
-
Recent advances in clay mineral-containing nanocomposite hydrogels.Soft Matter. 2015 Dec 28;11(48):9229-46. doi: 10.1039/c5sm01277e. Soft Matter. 2015. PMID: 26435008 Review.
-
Adsorption of nucleic Acid bases, ribose, and phosphate by some clay minerals.Life (Basel). 2015 Feb 27;5(1):637-50. doi: 10.3390/life5010637. Life (Basel). 2015. PMID: 25734235 Free PMC article. Review.
Cited by
-
Carbon and Nitrogen Mineralization in Relation to Soil Particle-Size Fractions after 32 Years of Chemical and Manure Application in a Continuous Maize Cropping System.PLoS One. 2016 Mar 31;11(3):e0152521. doi: 10.1371/journal.pone.0152521. eCollection 2016. PLoS One. 2016. PMID: 27031697 Free PMC article.
-
Compositional changes of crude oil SARA fractions due to biodegradation and adsorption on colloidal support such as clays using Iatroscan.Environ Sci Pollut Res Int. 2013 Sep;20(9):6445-54. doi: 10.1007/s11356-013-1635-8. Epub 2013 Apr 16. Environ Sci Pollut Res Int. 2013. PMID: 23589240
-
Red-Crowned Crane (Grus japonensis) Reproduction Was Improved by Inhibiting Mycotoxins with Montmorillonite in Feed.Toxins (Basel). 2020 Mar 18;12(3):191. doi: 10.3390/toxins12030191. Toxins (Basel). 2020. PMID: 32197419 Free PMC article.
-
Reduction of nitrogen compounds in oceanic basement and its implications for HCN formation and abiotic organic synthesis.Geochem Trans. 2009 Oct 22;10:9. doi: 10.1186/1467-4866-10-9. Geochem Trans. 2009. PMID: 19849830 Free PMC article.
-
Acid-Base Properties of Cis-Vacant Montmorillonite Edge Surfaces: A Combined First-Principles Molecular Dynamics and Surface Complexation Modeling Approach.Environ Sci Technol. 2023 Jan 10;57(3):1342-52. doi: 10.1021/acs.est.2c07171. Online ahead of print. Environ Sci Technol. 2023. PMID: 36627109 Free PMC article.
References
-
- Hendricks S B, Fry W H. Soil Sci. 1930;29:457–479.
-
- Sposito G. The Chemistry of Soils. New York: Oxford Univ. Press; 1989.
-
- Michalopoulos P, Aller R C. Science. 1995;270:614–617.
-
- McCauley S E, DePaolo D J. In: Tectonic Uplift and Climate Change. Ruddiman W F, editor. New York: Plenum; 1997. pp. 427–467.
LinkOut - more resources
Full Text Sources
Other Literature Sources