Identification of domains and residues within the epsilon subunit of eukaryotic translation initiation factor 2B (eIF2Bepsilon) required for guanine nucleotide exchange reveals a novel activation function promoted by eIF2B complex formation
- PMID: 10805739
- PMCID: PMC85753
- DOI: 10.1128/MCB.20.11.3965-3976.2000
Identification of domains and residues within the epsilon subunit of eukaryotic translation initiation factor 2B (eIF2Bepsilon) required for guanine nucleotide exchange reveals a novel activation function promoted by eIF2B complex formation
Abstract
Eukaryotic translation initiation factor 2B (eIF2B) is the guanine nucleotide exchange factor for protein synthesis initiation factor 2 (eIF2). Composed of five subunits, it converts eIF2 from a GDP-bound form to the active eIF2-GTP complex. This is a regulatory step of translation initiation. In vitro, eIF2B catalytic function can be provided by the largest (epsilon) subunit alone (eIF2Bepsilon). This activity is stimulated by complex formation with the other eIF2B subunits. We have analyzed the roles of different regions of eIF2Bepsilon in catalysis, in eIF2B complex formation, and in binding to eIF2 by characterizing mutations in the Saccharomyces cerevisiae gene encoding eIF2Bepsilon (GCD6) that impair the essential function of eIF2B. Our analysis of nonsense mutations indicates that the C terminus of eIF2Bepsilon (residues 518 to 712) is required for both catalytic activity and interaction with eIF2. In addition, missense mutations within this region impair the catalytic activity of eIF2Bepsilon without affecting its ability to bind eIF2. Internal, in-frame deletions within the N-terminal half of eIF2Bepsilon disrupt eIF2B complex formation without affecting the nucleotide exchange activity of eIF2Bepsilon alone. Finally, missense mutations identified within this region do not affect the catalytic activity of eIF2Bepsilon alone or its interactions with the other eIF2B subunits or with eIF2. Instead, these missense mutations act indirectly by impairing the enhancement of the rate of nucleotide exchange that results from complex formation between eIF2Bepsilon and the other eIF2B subunits. This suggests that the N-terminal region of eIF2Bepsilon is an activation domain that responds to eIF2B complex formation.
Figures
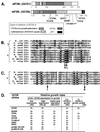
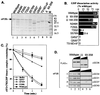
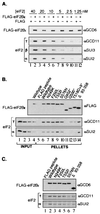
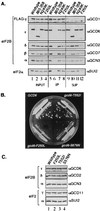
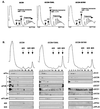
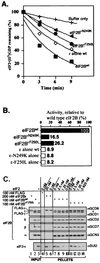
Similar articles
-
Conserved bipartite motifs in yeast eIF5 and eIF2Bepsilon, GTPase-activating and GDP-GTP exchange factors in translation initiation, mediate binding to their common substrate eIF2.EMBO J. 1999 Mar 15;18(6):1673-88. doi: 10.1093/emboj/18.6.1673. EMBO J. 1999. PMID: 10075937 Free PMC article.
-
Identification of a regulatory subcomplex in the guanine nucleotide exchange factor eIF2B that mediates inhibition by phosphorylated eIF2.Mol Cell Biol. 1996 Nov;16(11):6603-16. doi: 10.1128/MCB.16.11.6603. Mol Cell Biol. 1996. PMID: 8887689 Free PMC article.
-
The beta/Gcd7 subunit of eukaryotic translation initiation factor 2B (eIF2B), a guanine nucleotide exchange factor, is crucial for binding eIF2 in vivo.Mol Cell Biol. 2010 Nov;30(21):5218-33. doi: 10.1128/MCB.00265-10. Epub 2010 Aug 30. Mol Cell Biol. 2010. PMID: 20805354 Free PMC article.
-
Localization of the translational guanine nucleotide exchange factor eIF2B: a common theme for GEFs?Cell Cycle. 2006 Apr;5(7):678-80. doi: 10.4161/cc.5.7.2607. Epub 2006 Apr 1. Cell Cycle. 2006. PMID: 16582624 Review.
-
A new function and complexity for protein translation initiation factor eIF2B.Cell Cycle. 2014;13(17):2660-5. doi: 10.4161/15384101.2014.948797. Cell Cycle. 2014. PMID: 25486352 Free PMC article. Review.
Cited by
-
An eIF5/eIF2 complex antagonizes guanine nucleotide exchange by eIF2B during translation initiation.EMBO J. 2006 Oct 4;25(19):4537-46. doi: 10.1038/sj.emboj.7601339. Epub 2006 Sep 21. EMBO J. 2006. PMID: 16990799 Free PMC article.
-
Ectopic expression of eIF2Bepsilon in rat skeletal muscle rescues the sepsis-induced reduction in guanine nucleotide exchange activity and protein synthesis.Am J Physiol Endocrinol Metab. 2010 Aug;299(2):E241-8. doi: 10.1152/ajpendo.00151.2010. Epub 2010 May 18. Am J Physiol Endocrinol Metab. 2010. PMID: 20484009 Free PMC article.
-
Crystal structure of eukaryotic translation initiation factor 2B.Nature. 2016 Mar 3;531(7592):122-5. doi: 10.1038/nature16991. Epub 2016 Feb 22. Nature. 2016. PMID: 26901872
-
Fail-safe control of translation initiation by dissociation of eIF2α phosphorylated ternary complexes.Elife. 2017 Mar 18;6:e24542. doi: 10.7554/eLife.24542. Elife. 2017. PMID: 28315520 Free PMC article.
-
eIF2β is critical for eIF5-mediated GDP-dissociation inhibitor activity and translational control.Nucleic Acids Res. 2016 Nov 16;44(20):9698-9709. doi: 10.1093/nar/gkw657. Epub 2016 Jul 25. Nucleic Acids Res. 2016. PMID: 27458202 Free PMC article.
References
-
- Berlanga J J, Santoyo J, De Haro C. Characterization of a mammalian homolog of the GCN2 eukaryotic initiation factor 2alpha kinase. Eur J Biochem. 1999;265:754–762. - PubMed
-
- Boeke J D, LaCroute F, Fink G R. A positive selection for mutants lacking orotidine-5′-phosphate decarboxylase activity in yeast: 5-fluoro-orotic acid resistance. Mol Gen Genet. 1984;197:345–346. - PubMed
-
- Boguski M S, McCormick F. Proteins regulating Ras and its relatives. Nature. 1993;366:643–654. - PubMed
Publication types
MeSH terms
Substances
LinkOut - more resources
Full Text Sources
Molecular Biology Databases