Protoplasmic astrocytes in CA1 stratum radiatum occupy separate anatomical domains
- PMID: 11756501
- PMCID: PMC6757596
- DOI: 10.1523/JNEUROSCI.22-01-00183.2002
Protoplasmic astrocytes in CA1 stratum radiatum occupy separate anatomical domains
Abstract
Protoplasmic astrocytes are increasingly thought to interact extensively with neuronal elements in the brain and to influence their activity. Recent reports have also begun to suggest that physiologically, and perhaps functionally, diverse forms of these cells may be present in the CNS. Our current understanding of astrocyte form and distribution is based predominantly on studies that used the astrocytic marker glial fibrillary acidic protein (GFAP) and on studies using metal-impregnation techniques. The prevalent opinion, based on studies using these methods, is that astrocytic processes overlap extensively and primarily share the underlying neuropil. However, both of these techniques have serious shortcomings for visualizing the interactions among these structurally complex cells. In the present study, intracellular injection combined with immunohistochemistry for GFAP show that GFAP delineates only approximately 15% of the total volume of the astrocyte. As a result, GFAP-based images have led to incorrect conclusions regarding the interaction of processes of neighboring astrocytes. To investigate these interactions in detail, groups of adjacent protoplasmic astrocytes in the CA1 stratum radiatum were injected with fluorescent intracellular tracers of distinctive emissive wavelengths and analyzed using three-dimensional (3D) confocal analysis and electron microscopy. Our findings show that protoplasmic astrocytes establish primarily exclusive territories. The knowledge of how the complex morphology of protoplasmic astrocytes affects their 3D relationships with other astrocytes, oligodendroglia, neurons, and vasculature of the brain should have important implications for our understanding of nervous system function.
Figures
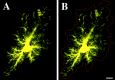
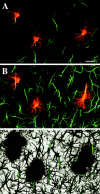
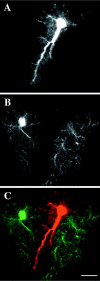
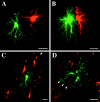
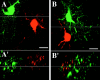
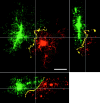
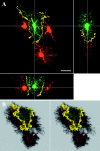
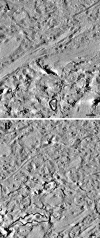
Similar articles
-
Maturation of astrocyte morphology and the establishment of astrocyte domains during postnatal hippocampal development.Int J Dev Neurosci. 2004 Apr;22(2):73-86. doi: 10.1016/j.ijdevneu.2003.12.008. Int J Dev Neurosci. 2004. PMID: 15036382
-
Spatial organization of NG2 glial cells and astrocytes in rat hippocampal CA1 region.Hippocampus. 2014 Apr;24(4):383-95. doi: 10.1002/hipo.22232. Epub 2013 Dec 13. Hippocampus. 2014. PMID: 24339242 Free PMC article.
-
Response of striatal astrocytes to neuronal deafferentation: an immunocytochemical and ultrastructural study.Neuroscience. 1994 Sep;62(2):425-39. doi: 10.1016/0306-4522(94)90377-8. Neuroscience. 1994. PMID: 7830889
-
Control of the phosphorylation of the astrocyte marker glial fibrillary acidic protein (GFAP) in the immature rat hippocampus by glutamate and calcium ions: possible key factor in astrocytic plasticity.Braz J Med Biol Res. 1997 Mar;30(3):325-38. doi: 10.1590/s0100-879x1997000300005. Braz J Med Biol Res. 1997. PMID: 9246230 Review.
-
Astrocytic Regulation of Neural Circuits Underlying Behaviors.Cells. 2021 Feb 1;10(2):296. doi: 10.3390/cells10020296. Cells. 2021. PMID: 33535587 Free PMC article. Review.
Cited by
-
The Time Course of Gene Expression during Reactive Gliosis in the Optic Nerve.PLoS One. 2013 Jun 27;8(6):e67094. doi: 10.1371/journal.pone.0067094. Print 2013. PLoS One. 2013. PMID: 23826199 Free PMC article.
-
LTP Induction Boosts Glutamate Spillover by Driving Withdrawal of Perisynaptic Astroglia.Neuron. 2020 Dec 9;108(5):919-936.e11. doi: 10.1016/j.neuron.2020.08.030. Epub 2020 Sep 24. Neuron. 2020. PMID: 32976770 Free PMC article.
-
Control of Ca2+ signals by astrocyte nanoscale morphology at tripartite synapses.Glia. 2022 Dec;70(12):2378-2391. doi: 10.1002/glia.24258. Epub 2022 Sep 13. Glia. 2022. PMID: 36097958 Free PMC article.
-
Astrocytes and developmental plasticity in fragile X.Neural Plast. 2012;2012:197491. doi: 10.1155/2012/197491. Epub 2012 Jul 11. Neural Plast. 2012. PMID: 22848847 Free PMC article. Review.
-
MCT4-mediated expression of EAAT1 is involved in the resistance to hypoxia injury in astrocyte-neuron co-cultures.Neurochem Res. 2015 Apr;40(4):818-28. doi: 10.1007/s11064-015-1532-2. Epub 2015 Feb 3. Neurochem Res. 2015. PMID: 25645447
References
-
- Araque A, Parpura V, Sanzgiri RP, Haydon PG. Tripartite synapses: glia, the unacknowledged partner. Trends Neurosci. 1999;22:208–215. - PubMed
-
- Belichenko PV, Dahlström A. Studies on the 3-dimensional architecture of dendritic spines and varicosities in human cortex by confocal laser scanning microscopy and Lucifer yellow microinjections. J Neurosci Methods. 1995;57:55–61. - PubMed
-
- Bignami A, Eng LF, Dahl D, Uyeda CT. Localization of the glial fibrillary acidic protein in astrocytes by immunofluorescence. Brain Res. 1972;43:429–435. - PubMed
-
- Bordey A, Sontheimer H. Passive glial cells, fact or artifact? J Membr Biol. 1998;166:213–222. - PubMed
-
- Buhl EH. Intracellular injection in fixed slices in combination with neuroanatomical tracing techniques and electron microscopy to determine multisynaptic pathways in the brain. Microsc Res Tech. 1993;24:15–30. - PubMed
Publication types
MeSH terms
Substances
Grants and funding
LinkOut - more resources
Full Text Sources
Other Literature Sources
Research Materials
Miscellaneous