Eukaryotic DNA ligases: structural and functional insights
- PMID: 18518823
- PMCID: PMC2933818
- DOI: 10.1146/annurev.biochem.77.061306.123941
Eukaryotic DNA ligases: structural and functional insights
Abstract
DNA ligases are required for DNA replication, repair, and recombination. In eukaryotes, there are three families of ATP-dependent DNA ligases. Members of the DNA ligase I and IV families are found in all eukaryotes, whereas DNA ligase III family members are restricted to vertebrates. These enzymes share a common catalytic region comprising a DNA-binding domain, a nucleotidyltransferase (NTase) domain, and an oligonucleotide/oligosaccharide binding (OB)-fold domain. The catalytic region encircles nicked DNA with each of the domains contacting the DNA duplex. The unique segments adjacent to the catalytic region of eukaryotic DNA ligases are involved in specific protein-protein interactions with a growing number of DNA replication and repair proteins. These interactions determine the specific cellular functions of the DNA ligase isozymes. In mammals, defects in DNA ligation have been linked with an increased incidence of cancer and neurodegeneration.
Figures
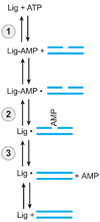
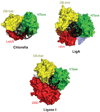
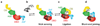
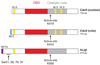
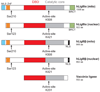
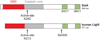
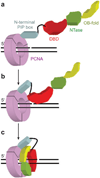
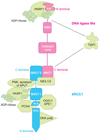
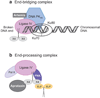
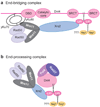
Similar articles
-
DNA ligases in the repair and replication of DNA.Mutat Res. 2000 Aug 30;460(3-4):301-18. doi: 10.1016/s0921-8777(00)00033-1. Mutat Res. 2000. PMID: 10946235 Review.
-
Structure-function analysis of the OB and latch domains of chlorella virus DNA ligase.J Biol Chem. 2011 Jun 24;286(25):22642-52. doi: 10.1074/jbc.M111.245399. Epub 2011 Apr 28. J Biol Chem. 2011. PMID: 21527793 Free PMC article.
-
DNA ligase I, the replicative DNA ligase.Subcell Biochem. 2012;62:327-41. doi: 10.1007/978-94-007-4572-8_17. Subcell Biochem. 2012. PMID: 22918593 Free PMC article. Review.
-
Human DNA ligase I completely encircles and partially unwinds nicked DNA.Nature. 2004 Nov 25;432(7016):473-8. doi: 10.1038/nature03082. Nature. 2004. PMID: 15565146
-
T4 DNA ligase structure reveals a prototypical ATP-dependent ligase with a unique mode of sliding clamp interaction.Nucleic Acids Res. 2018 Nov 2;46(19):10474-10488. doi: 10.1093/nar/gky776. Nucleic Acids Res. 2018. PMID: 30169742 Free PMC article.
Cited by
-
Rules of engagement for base excision repair in chromatin.J Cell Physiol. 2013 Feb;228(2):258-66. doi: 10.1002/jcp.24134. J Cell Physiol. 2013. PMID: 22718094 Free PMC article. Review.
-
Human DNA ligase III recognizes DNA ends by dynamic switching between two DNA-bound states.Biochemistry. 2010 Jul 27;49(29):6165-76. doi: 10.1021/bi100503w. Biochemistry. 2010. PMID: 20518483 Free PMC article.
-
Structural biology of DNA repair: spatial organisation of the multicomponent complexes of nonhomologous end joining.J Nucleic Acids. 2010 Aug 25;2010:621695. doi: 10.4061/2010/621695. J Nucleic Acids. 2010. PMID: 20862368 Free PMC article.
-
Structure-Function Analysis Reveals the Singularity of Plant Mitochondrial DNA Replication Components: A Mosaic and Redundant System.Plants (Basel). 2019 Nov 21;8(12):533. doi: 10.3390/plants8120533. Plants (Basel). 2019. PMID: 31766564 Free PMC article. Review.
-
Seed Longevity in Legumes: Deeper Insights Into Mechanisms and Molecular Perspectives.Front Plant Sci. 2022 Jul 27;13:918206. doi: 10.3389/fpls.2022.918206. eCollection 2022. Front Plant Sci. 2022. PMID: 35968115 Free PMC article.
References
Publication types
MeSH terms
Substances
Grants and funding
LinkOut - more resources
Full Text Sources
Other Literature Sources
Medical
Research Materials
Miscellaneous