Enhancer of polycomb1 acts on serum response factor to regulate skeletal muscle differentiation
- PMID: 19359245
- PMCID: PMC2713522
- DOI: 10.1074/jbc.M807725200
Enhancer of polycomb1 acts on serum response factor to regulate skeletal muscle differentiation
Abstract
Skeletal muscle differentiation is well regulated by a series of transcription factors. We reported previously that enhancer of polycomb1 (Epc1), a chromatin protein, can modulate skeletal muscle differentiation, although the mechanisms of this action have yet to be defined. Here we report that Epc1 recruits both serum response factor (SRF) and p300 to induce skeletal muscle differentiation. Epc1 interacted physically with SRF. Transfection of Epc1 to myoblast cells potentiated the SRF-induced expression of skeletal muscle-specific genes as well as multinucleation. Proximal CArG box in the skeletal alpha-actin promoter was responsible for the synergistic activation of the promoter-luciferase. Epc1 knockdown caused a decrease in the acetylation of histones associated with serum response element (SRE) of the skeletal alpha-actin promoter. The Epc1.SRF complex bound to the SRE, and the knockdown of Epc1 resulted in a decrease in SRF binding to the skeletal alpha-actin promoter. Epc1 recruited histone acetyltransferase activity, which was potentiated by cotransfection with p300 but abolished by si-p300. Epc1 directly bound to p300 in myoblast cells. Epc1+/- mice showed distortion of skeletal alpha-actin, and the isolated myoblasts from the mice had impaired muscle differentiation. These results suggest that Epc1 is required for skeletal muscle differentiation by recruiting both SRF and p300 to the SRE of muscle-specific gene promoters.
Figures
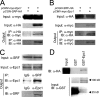
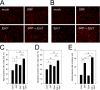
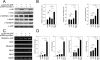
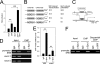
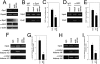
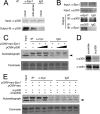
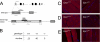
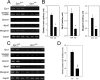
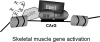
Similar articles
-
Ret finger protein inhibits muscle differentiation by modulating serum response factor and enhancer of polycomb1.Cell Death Differ. 2012 Jan;19(1):121-31. doi: 10.1038/cdd.2011.72. Epub 2011 Jun 3. Cell Death Differ. 2012. PMID: 21637294 Free PMC article.
-
Enhancer of polycomb1 lessens neointima formation by potentiation of myocardin-induced smooth muscle differentiation.Atherosclerosis. 2012 May;222(1):84-91. doi: 10.1016/j.atherosclerosis.2012.02.015. Epub 2012 Feb 18. Atherosclerosis. 2012. PMID: 22398275
-
Enhancer of polycomb1, a novel homeodomain only protein-binding partner, induces skeletal muscle differentiation.J Biol Chem. 2007 Mar 9;282(10):7700-9. doi: 10.1074/jbc.M611198200. Epub 2006 Dec 27. J Biol Chem. 2007. PMID: 17192267
-
Serum response factor: toggling between disparate programs of gene expression.J Mol Cell Cardiol. 2003 Jun;35(6):577-93. doi: 10.1016/s0022-2828(03)00110-x. J Mol Cell Cardiol. 2003. PMID: 12788374 Review.
-
Transcriptional mechanisms regulating skeletal muscle differentiation, growth and homeostasis.Nat Rev Mol Cell Biol. 2011 Jun;12(6):349-61. doi: 10.1038/nrm3118. Nat Rev Mol Cell Biol. 2011. PMID: 21602905 Review.
Cited by
-
MRTF-A regulates myoblast commitment to differentiation by targeting PAX7 during muscle regeneration.J Cell Mol Med. 2021 Sep;25(18):8645-8661. doi: 10.1111/jcmm.16820. Epub 2021 Aug 4. J Cell Mol Med. 2021. PMID: 34347392 Free PMC article.
-
Critical genomic regulation mediated by Enhancer of Polycomb.Curr Genet. 2018 Feb;64(1):147-154. doi: 10.1007/s00294-017-0742-3. Epub 2017 Sep 7. Curr Genet. 2018. PMID: 28884217 Free PMC article. Review.
-
Histone methyltransferase SETD3 regulates muscle differentiation.J Biol Chem. 2011 Oct 7;286(40):34733-42. doi: 10.1074/jbc.M110.203307. Epub 2011 Aug 8. J Biol Chem. 2011. PMID: 21832073 Free PMC article.
-
EPC1/2 regulate hematopoietic stem and progenitor cell proliferation by modulating H3 acetylation and DLST.iScience. 2024 Feb 17;27(3):109263. doi: 10.1016/j.isci.2024.109263. eCollection 2024 Mar 15. iScience. 2024. PMID: 38439957 Free PMC article.
-
Serum response factor promotes axon regeneration following spinal cord transection injury.Neural Regen Res. 2023 Sep;18(9):1956-1960. doi: 10.4103/1673-5374.367974. Neural Regen Res. 2023. PMID: 36926719 Free PMC article.
References
-
- Edmondson D. G., Olson E. N. ( 1993) J. Biol. Chem. 268, 755– 758 - PubMed
-
- McKinsey T. A., Zhang C. L., Olson E. N. ( 2001) Curr. Opin. Genet. Dev. 11, 497– 504 - PubMed
-
- Black B. L., Olson E. N. ( 1998) Annu. Rev. Cell Dev. Biol. 14, 167– 196 - PubMed
-
- Flück M., Booth F. W., Waxham M. N. ( 2000) Biochem. Biophys. Res. Commun. 270, 488– 494 - PubMed
Publication types
MeSH terms
Substances
LinkOut - more resources
Full Text Sources
Molecular Biology Databases
Miscellaneous