Responses of the ear to low frequency sounds, infrasound and wind turbines
- PMID: 20561575
- PMCID: PMC2923251
- DOI: 10.1016/j.heares.2010.06.007
Responses of the ear to low frequency sounds, infrasound and wind turbines
Abstract
Infrasonic sounds are generated internally in the body (by respiration, heartbeat, coughing, etc) and by external sources, such as air conditioning systems, inside vehicles, some industrial processes and, now becoming increasingly prevalent, wind turbines. It is widely assumed that infrasound presented at an amplitude below what is audible has no influence on the ear. In this review, we consider possible ways that low frequency sounds, at levels that may or may not be heard, could influence the function of the ear. The inner ear has elaborate mechanisms to attenuate low frequency sound components before they are transmitted to the brain. The auditory portion of the ear, the cochlea, has two types of sensory cells, inner hair cells (IHC) and outer hair cells (OHC), of which the IHC are coupled to the afferent fibers that transmit "hearing" to the brain. The sensory stereocilia ("hairs") on the IHC are "fluid coupled" to mechanical stimuli, so their responses depend on stimulus velocity and their sensitivity decreases as sound frequency is lowered. In contrast, the OHC are directly coupled to mechanical stimuli, so their input remains greater than for IHC at low frequencies. At very low frequencies the OHC are stimulated by sounds at levels below those that are heard. Although the hair cells in other sensory structures such as the saccule may be tuned to infrasonic frequencies, auditory stimulus coupling to these structures is inefficient so that they are unlikely to be influenced by airborne infrasound. Structures that are involved in endolymph volume regulation are also known to be influenced by infrasound, but their sensitivity is also thought to be low. There are, however, abnormal states in which the ear becomes hypersensitive to infrasound. In most cases, the inner ear's responses to infrasound can be considered normal, but they could be associated with unfamiliar sensations or subtle changes in physiology. This raises the possibility that exposure to the infrasound component of wind turbine noise could influence the physiology of the ear.
Copyright (c) 2010 Elsevier B.V. All rights reserved.
Figures
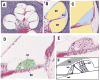
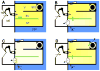
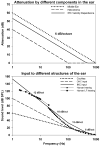
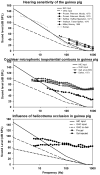
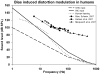
Similar articles
-
Infrasonic hearing in birds: a review of audiometry and hypothesized structure-function relationships.Biol Rev Camb Philos Soc. 2020 Aug;95(4):1036-1054. doi: 10.1111/brv.12596. Epub 2020 Mar 31. Biol Rev Camb Philos Soc. 2020. PMID: 32237036 Review.
-
Large endolymphatic potentials from low-frequency and infrasonic tones in the guinea pig.J Acoust Soc Am. 2013 Mar;133(3):1561-71. doi: 10.1121/1.4789005. J Acoust Soc Am. 2013. PMID: 23464026
-
Effect of infrasound on cochlear damage from exposure to a 4 kHz octave band of noise.Hear Res. 2007 Mar;225(1-2):128-38. doi: 10.1016/j.heares.2007.01.016. Epub 2007 Jan 19. Hear Res. 2007. PMID: 17300889 Free PMC article.
-
'Wind turbine syndrome': fact or fiction?J Laryngol Otol. 2013 Mar;127(3):222-6. doi: 10.1017/S0022215112002964. J Laryngol Otol. 2013. PMID: 23331380 Review.
-
Symptoms intuitively associated with wind turbine infrasound.Environ Res. 2021 Jan;192:110360. doi: 10.1016/j.envres.2020.110360. Epub 2020 Oct 22. Environ Res. 2021. PMID: 33131679
Cited by
-
Wind turbines and human health.Front Public Health. 2014 Jun 19;2:63. doi: 10.3389/fpubh.2014.00063. eCollection 2014. Front Public Health. 2014. PMID: 24995266 Free PMC article. Review.
-
Distortion-Product Otoacoustic Emission Measured Below 300 Hz in Normal-Hearing Human Subjects.J Assoc Res Otolaryngol. 2017 Apr;18(2):197-208. doi: 10.1007/s10162-016-0600-x. Epub 2016 Nov 21. J Assoc Res Otolaryngol. 2017. PMID: 27873084 Free PMC article.
-
Health effects and wind turbines: a review of the literature.Environ Health. 2011 Sep 14;10:78. doi: 10.1186/1476-069X-10-78. Environ Health. 2011. PMID: 21914211 Free PMC article. Review.
-
Altered cortical and subcortical connectivity due to infrasound administered near the hearing threshold - Evidence from fMRI.PLoS One. 2017 Apr 12;12(4):e0174420. doi: 10.1371/journal.pone.0174420. eCollection 2017. PLoS One. 2017. PMID: 28403175 Free PMC article.
-
Asymmetric Flankers in Comodulation Masking Release.J Audiol Otol. 2019 Jan;23(1):27-32. doi: 10.7874/jao.2018.00192. Epub 2018 Aug 22. J Audiol Otol. 2019. PMID: 30126261 Free PMC article.
References
-
- Bian L, Linhardt EE, Chertoff ME. Cochlear hysteresis: observation with low-frequency modulated distortion product otoacoustic emissions. J Acoust Soc Am. 2004;115:2159–2172. - PubMed
-
- British Wind Energy Association. 2010. http://www.bwea.com/ref/noise.html.
-
- Brown MC. Antidromic responses of single units from the spiral ganglion. J Neurophysiol. 1994;71:1835–1847. - PubMed
Publication types
MeSH terms
Grants and funding
LinkOut - more resources
Full Text Sources
Medical