Flavokawain B, the hepatotoxic constituent from kava root, induces GSH-sensitive oxidative stress through modulation of IKK/NF-kappaB and MAPK signaling pathways
- PMID: 20696856
- PMCID: PMC2992378
- DOI: 10.1096/fj.10-163311
Flavokawain B, the hepatotoxic constituent from kava root, induces GSH-sensitive oxidative stress through modulation of IKK/NF-kappaB and MAPK signaling pathways
Abstract
Kava (Piper methysticum Foster, Piperaceae) organic solvent-extract has been used to treat mild to moderate anxiety, insomnia, and muscle fatigue in Western countries, leading to its emergence as one of the 10 best-selling herbal preparations. However, several reports of severe hepatotoxicity in kava consumers led the U.S. Food and Drug Administration and authorities in Europe to restrict sales of kava-containing products. Herein we demonstrate that flavokawain B (FKB), a chalcone from kava root, is a potent hepatocellular toxin, inducing cell death in HepG2 (LD(50)=15.3 ± 0.2 μM) and L-02 (LD(50)=32 μM) cells. Hepatocellular toxicity of FKB is mediated by induction of oxidative stress, depletion of reduced glutathione (GSH), inhibition of IKK activity leading to NF-κB transcriptional blockade, and constitutive TNF-α-independent activation of mitogen-activated protein kinase (MAPK) signaling pathways, namely, ERK, p38, and JNK. We further demonstrate by noninvasive bioluminescence imaging that oral consumption of FKB leads to inhibition of hepatic NF-κB transcriptional activity in vivo and severe liver damage. Surprisingly, replenishment with exogenous GSH normalizes both TNF-α-dependent NF-κB as well as MAPK signaling and rescues hepatocytes from FKB-induced death. Our data identify FKB as a potent GSH-sensitive hepatotoxin, levels of which should be specifically monitored and controlled in kava-containing herb products.
Figures
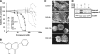
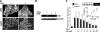
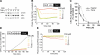
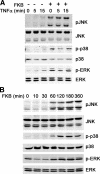
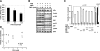
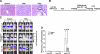
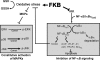
Similar articles
-
Flavokawains A and B from kava (Piper methysticum) activate heat shock and antioxidant responses and protect against hydrogen peroxide-induced cell death in HepG2 hepatocytes.Pharm Biol. 2016 Sep;54(9):1503-12. doi: 10.3109/13880209.2015.1107104. Epub 2016 Jan 20. Pharm Biol. 2016. PMID: 26789234 Free PMC article.
-
Inhibition of TNFalpha-induced activation of nuclear factor kappaB by kava (Piper methysticum) derivatives.Biochem Pharmacol. 2006 Apr 14;71(8):1206-18. doi: 10.1016/j.bcp.2005.12.032. Epub 2006 Feb 7. Biochem Pharmacol. 2006. PMID: 16464438
-
Glutathione depletion down-regulates tumor necrosis factor alpha-induced NF-kappaB activity via IkappaB kinase-dependent and -independent mechanisms.J Biol Chem. 2007 Oct 5;282(40):29470-81. doi: 10.1074/jbc.M706145200. Epub 2007 Aug 9. J Biol Chem. 2007. PMID: 17690092
-
Kava as a Clinical Nutrient: Promises and Challenges.Nutrients. 2020 Oct 5;12(10):3044. doi: 10.3390/nu12103044. Nutrients. 2020. PMID: 33027883 Free PMC article. Review.
-
Toxicity of kava kava.J Environ Sci Health C Environ Carcinog Ecotoxicol Rev. 2008 Jan-Mar;26(1):89-112. doi: 10.1080/10590500801907407. J Environ Sci Health C Environ Carcinog Ecotoxicol Rev. 2008. PMID: 18322868 Free PMC article. Review.
Cited by
-
In Silico Discovery of Potential Uridine-Cytidine Kinase 2 Inhibitors from the Rhizome of Alpinia mutica.Molecules. 2016 Apr 8;21(4):417. doi: 10.3390/molecules21040417. Molecules. 2016. PMID: 27070566 Free PMC article.
-
A UHPLC-UV Method Development and Validation for Determining Kavalactones and Flavokavains in Piper methysticum (Kava).Molecules. 2019 Mar 30;24(7):1245. doi: 10.3390/molecules24071245. Molecules. 2019. PMID: 30934989 Free PMC article.
-
Dihydro-5,6-dehydrokavain (DDK) from Alpinia zerumbet: Its Isolation, Synthesis, and Characterization.Molecules. 2015 Sep 9;20(9):16306-19. doi: 10.3390/molecules200916306. Molecules. 2015. PMID: 26370954 Free PMC article. Review.
-
Toxicity Potential of Nutraceuticals.Methods Mol Biol. 2025;2834:197-230. doi: 10.1007/978-1-0716-4003-6_10. Methods Mol Biol. 2025. PMID: 39312167
-
Flavokawain B, a kava chalcone, inhibits growth of human osteosarcoma cells through G2/M cell cycle arrest and apoptosis.Mol Cancer. 2013 Jun 10;12:55. doi: 10.1186/1476-4598-12-55. Mol Cancer. 2013. PMID: 23764122 Free PMC article.
References
-
- Singh Y. N. (1992) Kava: an overview. J. Ethnopharmacol. 37, 13–45 - PubMed
-
- Singh Y., Blumenthal M. (1997) Kava: An overview. HerbalGram 39, 33–47
-
- Centers for Disease Control and Prevention (2003) CDC Advisory. Hepatic toxicity possibly associated with kava-containing products: United States, Germany, and Switzerland, 1999–2002. JAMA 289, 36–37 - PubMed
-
- MMWR 2002. Hepatic toxicity possibly associated with kava-containing products: United States, Germany, and Switzerland, 1999–2002. Morb. Mortal. Wkly. Rep. 51, 1065–1067 - PubMed
-
- Gow P. J., Connelly N. J., Hill R. L., Crowley P., Angus P. W. (2003) Fatal fulminant hepatic failure induced by a natural therapy containing kava. Med. J. Aust. 178, 442–443 - PubMed
Publication types
MeSH terms
Substances
Grants and funding
LinkOut - more resources
Full Text Sources
Other Literature Sources
Research Materials
Miscellaneous