Regulation of blood pressure and salt homeostasis by endothelin
- PMID: 21248162
- PMCID: PMC3236687
- DOI: 10.1152/physrev.00060.2009
Regulation of blood pressure and salt homeostasis by endothelin
Abstract
Endothelin (ET) peptides and their receptors are intimately involved in the physiological control of systemic blood pressure and body Na homeostasis, exerting these effects through alterations in a host of circulating and local factors. Hormonal systems affected by ET include natriuretic peptides, aldosterone, catecholamines, and angiotensin. ET also directly regulates cardiac output, central and peripheral nervous system activity, renal Na and water excretion, systemic vascular resistance, and venous capacitance. ET regulation of these systems is often complex, sometimes involving opposing actions depending on which receptor isoform is activated, which cells are affected, and what other prevailing factors exist. A detailed understanding of this system is important; disordered regulation of the ET system is strongly associated with hypertension and dysregulated extracellular fluid volume homeostasis. In addition, ET receptor antagonists are being increasingly used for the treatment of a variety of diseases; while demonstrating benefit, these agents also have adverse effects on fluid retention that may substantially limit their clinical utility. This review provides a detailed analysis of how the ET system is involved in the control of blood pressure and Na homeostasis, focusing primarily on physiological regulation with some discussion of the role of the ET system in hypertension.
Figures
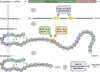
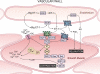
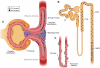
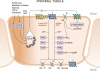
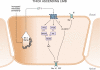
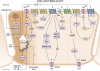
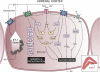
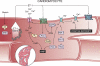
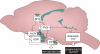
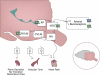
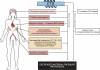
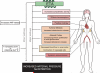
Similar articles
-
Role of collecting duct endothelin in control of renal function and blood pressure.Am J Physiol Regul Integr Comp Physiol. 2013 Oct 1;305(7):R659-68. doi: 10.1152/ajpregu.00345.2013. Epub 2013 Aug 28. Am J Physiol Regul Integr Comp Physiol. 2013. PMID: 23986358 Free PMC article. Review.
-
Endothelin as a regulator of cardiovascular function in health and disease.J Hypertens. 1998 Aug;16(8):1081-98. doi: 10.1097/00004872-199816080-00001. J Hypertens. 1998. PMID: 9794709 Review.
-
Participation of renal and circulating endothelin in salt-sensitive essential hypertension.J Hum Hypertens. 2002 Jul;16(7):459-67. doi: 10.1038/sj.jhh.1001419. J Hum Hypertens. 2002. PMID: 12080429 Review.
-
Endothelins as cardiovascular peptides.Am J Nephrol. 1996;16(3):246-51. doi: 10.1159/000169004. Am J Nephrol. 1996. PMID: 8739884 Review.
-
Family history and pathophysiological mechanisms of primary hypertension. Studies in non-hypertensive young men with positive and negative family histories of hypertension.Scand J Urol Nephrol Suppl. 1991;134:1-75. Scand J Urol Nephrol Suppl. 1991. PMID: 1887213
Cited by
-
Endothelin, kidney disease, and hypertension.Hypertension. 2013 Jun;61(6):1142-5. doi: 10.1161/HYPERTENSIONAHA.113.00595. Epub 2013 Apr 22. Hypertension. 2013. PMID: 23608655 Free PMC article. Review. No abstract available.
-
Activation of purinergic receptors (P2) in the renal medulla promotes endothelin-dependent natriuresis in male rats.Am J Physiol Renal Physiol. 2016 Aug 1;311(2):F260-7. doi: 10.1152/ajprenal.00090.2016. Epub 2016 May 25. Am J Physiol Renal Physiol. 2016. PMID: 27226106 Free PMC article.
-
Induction of renal tumor necrosis factor-α and other autacoids and the beneficial effects of hypertonic saline in acute decompensated heart failure.Am J Physiol Renal Physiol. 2021 Jun 1;320(6):F1159-F1164. doi: 10.1152/ajprenal.00686.2020. Epub 2021 May 10. Am J Physiol Renal Physiol. 2021. PMID: 33969695 Free PMC article.
-
Endothelin antagonists for diabetic and non-diabetic chronic kidney disease.Br J Clin Pharmacol. 2013 Oct;76(4):573-9. doi: 10.1111/bcp.12064. Br J Clin Pharmacol. 2013. PMID: 23228194 Free PMC article. Review.
-
Endothelin-3 Suppresses Luteinizing Hormone Receptor Expression by Regulating the cAMP-PKA Pathway in Hen Granulosa Cells.Curr Issues Mol Biol. 2024 Jul 23;46(8):7832-7845. doi: 10.3390/cimb46080464. Curr Issues Mol Biol. 2024. PMID: 39194681 Free PMC article.
References
-
- Aarnio P, McGregor CG, Miller V. Autonomic modulation of contractions to endothelin-1 in canine coronary arteries. Hypertension. 1993;21:680–686. - PubMed
-
- Abassi Z, Ellahham S, Winaver J, Hoffman A. The intrarenal endothelin system and hypertension. News Physiol Sci. 2001;16:152–156. - PubMed
-
- Abassi ZA, Tate JE, Golomb E, Keiser HR. Role of neutral endopeptidase in the metabolism of endothelin. Hypertension. 1992;20:89–95. - PubMed
-
- Ackermann M, Ritthaler T, Riegger G, Kurtz A, Kramer BK. Endothelin inhibits cAMP-induced renal release from isolated renal juxtaglomerular cells. J Cardiovasc Pharmacol. 1995:S135–S137. 26. - PubMed
Publication types
MeSH terms
Substances
Grants and funding
- P01 HL069999-09/HL/NHLBI NIH HHS/United States
- R01 HL060653-10/HL/NHLBI NIH HHS/United States
- R01 HL079102-05/HL/NHLBI NIH HHS/United States
- HL-60653/HL/NHLBI NIH HHS/United States
- HL-74167/HL/NHLBI NIH HHS/United States
- HL-95819/HL/NHLBI NIH HHS/United States
- DK-44628/DK/NIDDK NIH HHS/United States
- R01 HL098135-02/HL/NHLBI NIH HHS/United States
- R01 HL060653/HL/NHLBI NIH HHS/United States
- DK-96392/DK/NIDDK NIH HHS/United States
- P01 HL069999/HL/NHLBI NIH HHS/United States
- R01 DK069392/DK/NIDDK NIH HHS/United States
- R01 HL095819/HL/NHLBI NIH HHS/United States
- R01 DK044628-17/DK/NIDDK NIH HHS/United States
- HL-69999/HL/NHLBI NIH HHS/United States
- P01 HL074167/HL/NHLBI NIH HHS/United States
- R01 HL079102/HL/NHLBI NIH HHS/United States
- R01 DK069392-04/DK/NIDDK NIH HHS/United States
- R01 DK044628/DK/NIDDK NIH HHS/United States
- R01 HL098135/HL/NHLBI NIH HHS/United States
- R29 DK044628/DK/NIDDK NIH HHS/United States
- HL-79102/HL/NHLBI NIH HHS/United States
LinkOut - more resources
Full Text Sources
Other Literature Sources
Medical
Molecular Biology Databases