The articulation of sauropod necks: methodology and mythology
- PMID: 24205266
- PMCID: PMC3812995
- DOI: 10.1371/journal.pone.0078572
The articulation of sauropod necks: methodology and mythology
Abstract
Sauropods are often imagined to have held their heads high atop necks that ascended in a sweeping curve that was formed either intrinsically because of the shape of their vertebrae, or behaviorally by lifting the head, or both. Their necks are also popularly depicted in life with poses suggesting avian flexibility. The grounds for such interpretations are examined in terms of vertebral osteology, inferences about missing soft tissues, intervertebral flexibility, and behavior. Osteologically, the pronounced opisthocoely and conformal central and zygapophyseal articular surfaces strongly constrain the reconstruction of the cervical vertebral column. The sauropod cervico-dorsal vertebral column is essentially straight, in contrast to the curvature exhibited in those extant vertebrates that naturally hold their heads above rising necks. Regarding flexibility, extant vertebrates with homologous articular geometries preserve a degree of zygapophyseal overlap at the limits of deflection, a constraint that is further restricted by soft tissues. Sauropod necks, if similarly constrained, were capable of sweeping out large feeding surfaces, yet much less capable of retracting the head to explore the enclosed volume in an avian manner. Behaviorally, modern vertebrates generally assume characteristic neck postures which are close to the intrinsic curvature of the undeflected neck. With the exception of some vertebrates that can retract their heads to balance above their shoulders at rest (e.g., felids, lagomorphs, and some ratites), the undeflected neck generally predicts the default head height at rest and during locomotion.
Conflict of interest statement
Figures
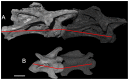
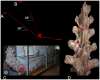
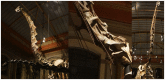
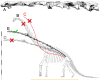
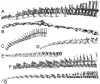
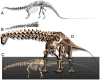
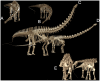
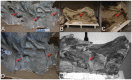
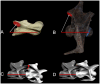
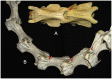
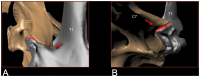
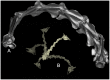
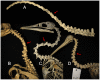
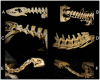
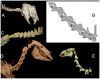
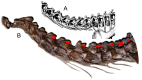
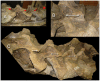
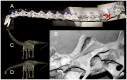
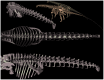
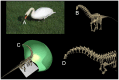
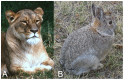
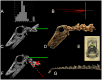
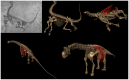
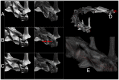
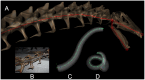
Similar articles
-
The effect of intervertebral cartilage on neutral posture and range of motion in the necks of sauropod dinosaurs.PLoS One. 2013 Oct 30;8(10):e78214. doi: 10.1371/journal.pone.0078214. eCollection 2013. PLoS One. 2013. PMID: 24205163 Free PMC article.
-
Torsion and bending in the neck and tail of sauropod dinosaurs and the function of cervical ribs: insights from functional morphology and biomechanics.PLoS One. 2013 Oct 30;8(10):e78574. doi: 10.1371/journal.pone.0078574. eCollection 2013. PLoS One. 2013. PMID: 24205268 Free PMC article.
-
Neck posture and feeding habits of two jurassic sauropod dinosaurs.Science. 1999 Apr 30;284(5415):798-800. doi: 10.1126/science.284.5415.798. Science. 1999. PMID: 10221910
-
Biology of the sauropod dinosaurs: the evolution of gigantism.Biol Rev Camb Philos Soc. 2011 Feb;86(1):117-55. doi: 10.1111/j.1469-185X.2010.00137.x. Biol Rev Camb Philos Soc. 2011. PMID: 21251189 Free PMC article. Review.
-
Dinosaur biomechanics.Proc Biol Sci. 2006 Aug 7;273(1596):1849-55. doi: 10.1098/rspb.2006.3532. Proc Biol Sci. 2006. PMID: 16822743 Free PMC article. Review.
Cited by
-
High browsing skeletal adaptations in Spinophorosaurus reveal an evolutionary innovation in sauropod dinosaurs.Sci Rep. 2020 Apr 20;10(1):6638. doi: 10.1038/s41598-020-63439-0. Sci Rep. 2020. PMID: 32313018 Free PMC article.
-
A method for deducing neck mobility in plesiosaurs, using the exceptionally preserved Nichollssaura borealis.R Soc Open Sci. 2018 Aug 1;5(8):172307. doi: 10.1098/rsos.172307. eCollection 2018 Aug. R Soc Open Sci. 2018. PMID: 30224996 Free PMC article.
-
Functional cervicothoracic boundary modified by anatomical shifts in the neck of giraffes.R Soc Open Sci. 2016 Feb 3;3(2):150604. doi: 10.1098/rsos.150604. eCollection 2016 Feb. R Soc Open Sci. 2016. PMID: 26998330 Free PMC article.
-
Multibody analysis and soft tissue strength refute supersonic dinosaur tail.Sci Rep. 2022 Dec 8;12(1):19245. doi: 10.1038/s41598-022-21633-2. Sci Rep. 2022. PMID: 36482175 Free PMC article.
-
Neck mobility in the Jurassic plesiosaur Cryptoclidus eurymerus: finite element analysis as a new approach to understanding the cervical skeleton in fossil vertebrates.PeerJ. 2019 Nov 6;7:e7658. doi: 10.7717/peerj.7658. eCollection 2019. PeerJ. 2019. PMID: 31720095 Free PMC article.
References
-
- Reisdorf AG, Wuttke M (2012) Re-evaluating Moodie's Opisthotonic- Posture Hypothesis in Fossil Vertebrates Part I: Reptiles – the taphonomy of the bipedal dinosaurs Compsognathus longipes and Juravenator starki from the Solnhofen Archipelago (Jurassic, Germany). Palaeobiodiversity and Palaeoenvironments 92: 119–168 10.1007/s12549-011-0068-y - DOI
-
- Tornier G (1909) Wie war der Diplodocus carnegii wirklich gebaut? Sitzungsberichte der Gesellschaft Naturforschender Freunde zu Berlin 1909-4: 193–209.
-
- Hay OP (1910) On the manner of locomotion of the dinosaurs, especially Diplodocus, with remarks on the origin of the birds. Proceedings of the Washington Academy of Sciences. 12: 1–25.
-
- Wiman C (1929) Die Kriede-dinosaurier aus Shantung. Palaeontologica Sinica (Series C) 6: 1–67.
-
- Paul G (1998) Differing bipedal and tripodal feeding modes in sauropods. Journal of Vertebrate Paleontology 69: 70A.
Publication types
MeSH terms
Grants and funding
LinkOut - more resources
Full Text Sources
Other Literature Sources