Microbially induced sedimentary structures recording an ancient ecosystem in the ca. 3.48 billion-year-old Dresser Formation, Pilbara, Western Australia
- PMID: 24205812
- PMCID: PMC3870916
- DOI: 10.1089/ast.2013.1030
Microbially induced sedimentary structures recording an ancient ecosystem in the ca. 3.48 billion-year-old Dresser Formation, Pilbara, Western Australia
Abstract
Microbially induced sedimentary structures (MISS) result from the response of microbial mats to physical sediment dynamics. MISS are cosmopolitan and found in many modern environments, including shelves, tidal flats, lagoons, riverine shores, lakes, interdune areas, and sabkhas. The structures record highly diverse communities of microbial mats and have been reported from numerous intervals in the geological record up to 3.2 billion years (Ga) old. This contribution describes a suite of MISS from some of the oldest well-preserved sedimentary rocks in the geological record, the early Archean (ca. 3.48 Ga) Dresser Formation, Western Australia. Outcrop mapping at the meter to millimeter scale defined five sub-environments characteristic of an ancient coastal sabkha. These sub-environments contain associations of distinct macroscopic and microscopic MISS. Macroscopic MISS include polygonal oscillation cracks and gas domes, erosional remnants and pockets, and mat chips. Microscopic MISS comprise tufts, sinoidal structures, and laminae fabrics; the microscopic laminae are composed of primary carbonaceous matter, pyrite, and hematite, plus trapped and bound grains. Identical suites of MISS occur in equivalent environmental settings through the entire subsequent history of Earth including the present time. This work extends the geological record of MISS by almost 300 million years. Complex mat-forming microbial communities likely existed almost 3.5 billion years ago.
Figures
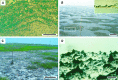
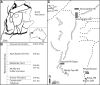
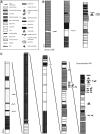
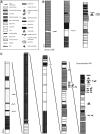
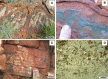
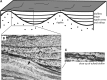
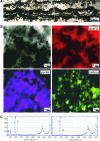
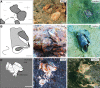
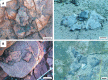
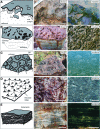
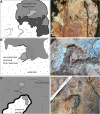
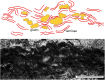
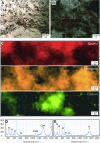
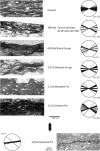

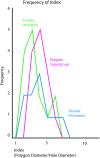
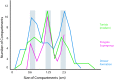
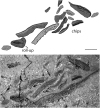
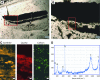
Similar articles
-
Ancient sedimentary structures in the <3.7 Ga Gillespie Lake Member, Mars, that resemble macroscopic morphology, spatial associations, and temporal succession in terrestrial microbialites.Astrobiology. 2015 Feb;15(2):169-92. doi: 10.1089/ast.2014.1218. Epub 2014 Dec 11. Astrobiology. 2015. PMID: 25495393
-
An actualistic perspective into Archean worlds - (cyano-)bacterially induced sedimentary structures in the siliciclastic Nhlazatse Section, 2.9 Ga Pongola Supergroup, South Africa.Geobiology. 2008 Jan;6(1):5-20. doi: 10.1111/j.1472-4669.2007.00118.x. Geobiology. 2008. PMID: 18380882
-
Interpreting an Archaean paleoenvironment through 3D imagery of microbialites.Geobiology. 2024 May-Jun;22(3):e12601. doi: 10.1111/gbi.12601. Geobiology. 2024. PMID: 38725142
-
Community living long before man: fossil and living microbial mats and early life.Sci Total Environ. 1986;56:379-97. doi: 10.1016/0048-9697(86)90342-6. Sci Total Environ. 1986. PMID: 11542059 Review.
-
Archean microfossils: a reappraisal of early life on Earth.Res Microbiol. 2003 Nov;154(9):611-7. doi: 10.1016/j.resmic.2003.08.006. Res Microbiol. 2003. PMID: 14596897 Review.
Cited by
-
Viscoelasticity of biofilms and their recalcitrance to mechanical and chemical challenges.FEMS Microbiol Rev. 2015 Mar;39(2):234-45. doi: 10.1093/femsre/fuu008. Epub 2015 Feb 2. FEMS Microbiol Rev. 2015. PMID: 25725015 Free PMC article. Review.
-
Setting the geological scene for the origin of life and continuing open questions about its emergence.Front Astron Space Sci. 2023 Jan 5;9:1095701. doi: 10.3389/fspas.2022.1095701. Front Astron Space Sci. 2023. PMID: 38274407 Free PMC article.
-
Ancient Diseases in Vertebrates: Tumours through the Ages.Animals (Basel). 2024 May 15;14(10):1474. doi: 10.3390/ani14101474. Animals (Basel). 2024. PMID: 38791691 Free PMC article. Review.
-
Depthwise microbiome and isotopic profiling of a moderately saline microbial mat in a solar saltern.Sci Rep. 2020 Nov 26;10(1):20686. doi: 10.1038/s41598-020-77622-w. Sci Rep. 2020. PMID: 33244085 Free PMC article.
-
Studying the Human Microbiota: Advances in Understanding the Fundamentals, Origin, and Evolution of Biological Timekeeping.Int J Mol Sci. 2023 Nov 10;24(22):16169. doi: 10.3390/ijms242216169. Int J Mol Sci. 2023. PMID: 38003359 Free PMC article. Review.
References
-
- Allwood A.C., Walter M., Kamber B., Marshall C., and Burch I. (2006) Stromatolite reef from the early Archaean era of Australia. Nature 441:714–718 - PubMed
-
- Allwood A.C., Walter M.R., Burch I.W., and Kamber B.S. (2007) 3.43 billion-year-old stromatolite reef from the Pilbara Craton of Western Australia: ecosystem-scale insights to early life on Earth. Precambrian Res 158:198–227
-
- Allwood A.C., Kamber B.S., Walter M.R., Burch I.W., and Kanik I. (2010) Trace elements record depositional history of an Early Archean stromatolitic carbonate platform Chem Geol 270:148–163
-
- Australian Stratigraphic Units Database (2012) Dresser Formation. Geoscience Australia, Commonwealth of Australia, Canberra, Australia: Available online at http://dbforms.ga.gov.au/pls/www/geodx.strat_units.sch_full?wher=stratno...
Publication types
MeSH terms
LinkOut - more resources
Full Text Sources
Other Literature Sources