Crystal structure of the human primase
- PMID: 25550159
- PMCID: PMC4342476
- DOI: 10.1074/jbc.M114.624742
Crystal structure of the human primase
Abstract
DNA replication in bacteria and eukaryotes requires the activity of DNA primase, a DNA-dependent RNA polymerase that lays short RNA primers for DNA polymerases. Eukaryotic and archaeal primases are heterodimers consisting of small catalytic and large accessory subunits, both of which are necessary for RNA primer synthesis. Understanding of RNA synthesis priming in eukaryotes is currently limited due to the lack of crystal structures of the full-length primase and its complexes with substrates in initiation and elongation states. Here we report the crystal structure of the full-length human primase, revealing the precise overall organization of the enzyme, the relative positions of its functional domains, and the mode of its interaction with modeled DNA and RNA. The structure indicates that the dramatic conformational changes in primase are necessary to accomplish the initiation and then elongation of RNA synthesis. The presence of a long linker between the N- and C-terminal domains of p58 provides the structural basis for the bulk of enzyme's conformational flexibility. Deletion of most of this linker affected the initiation and elongation steps of the primer synthesis.
Keywords: Crystal Structure; DNA Primase; DNA Replication; Iron-Sulfur Protein; Protein Complex; Zinc.
© 2015 by The American Society for Biochemistry and Molecular Biology, Inc.
Figures
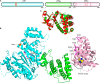
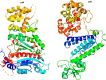
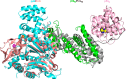
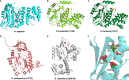
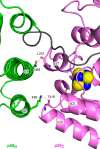
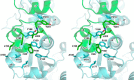
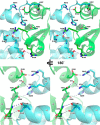
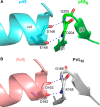
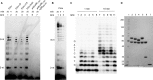
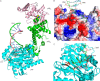
Similar articles
-
Crystal structure of the C-terminal domain of human DNA primase large subunit: implications for the mechanism of the primase-polymerase α switch.Cell Cycle. 2011 Mar 15;10(6):926-31. doi: 10.4161/cc.10.6.15010. Epub 2011 Mar 15. Cell Cycle. 2011. PMID: 21346410 Free PMC article.
-
Insight into the Human DNA Primase Interaction with Template-Primer.J Biol Chem. 2016 Feb 26;291(9):4793-802. doi: 10.1074/jbc.M115.704064. Epub 2015 Dec 28. J Biol Chem. 2016. PMID: 26710848 Free PMC article.
-
Insights into eukaryotic primer synthesis from structures of the p48 subunit of human DNA primase.J Mol Biol. 2014 Feb 6;426(3):558-69. doi: 10.1016/j.jmb.2013.11.007. Epub 2013 Nov 13. J Mol Biol. 2014. PMID: 24239947 Free PMC article.
-
The Pol α-primase complex.Subcell Biochem. 2012;62:157-69. doi: 10.1007/978-94-007-4572-8_9. Subcell Biochem. 2012. PMID: 22918585 Review.
-
Recent advances in understanding bacterial and archaeoeukaryotic primases.Curr Opin Struct Biol. 2019 Dec;59:159-167. doi: 10.1016/j.sbi.2019.08.004. Epub 2019 Oct 1. Curr Opin Struct Biol. 2019. PMID: 31585372 Review.
Cited by
-
The [4Fe4S] cluster of human DNA primase functions as a redox switch using DNA charge transport.Science. 2017 Feb 24;355(6327):eaag1789. doi: 10.1126/science.aag1789. Science. 2017. PMID: 28232525 Free PMC article.
-
Divalent ions attenuate DNA synthesis by human DNA polymerase α by changing the structure of the template/primer or by perturbing the polymerase reaction.DNA Repair (Amst). 2016 Jul;43:24-33. doi: 10.1016/j.dnarep.2016.05.017. Epub 2016 May 12. DNA Repair (Amst). 2016. PMID: 27235627 Free PMC article.
-
Comparison of the Proteome of Huh7 Cells Transfected with Hepatitis B Virus Subgenotype A1, with or without G1862T.Curr Issues Mol Biol. 2024 Jul 4;46(7):7032-7047. doi: 10.3390/cimb46070419. Curr Issues Mol Biol. 2024. PMID: 39057060 Free PMC article.
-
Telomere C-Strand Fill-In Machinery: New Insights into the Human CST-DNA Polymerase Alpha-Primase Structures and Functions.Subcell Biochem. 2024;104:73-100. doi: 10.1007/978-3-031-58843-3_5. Subcell Biochem. 2024. PMID: 38963484 Review.
-
The monomeric archaeal primase from Nanoarchaeum equitans harbours the features of heterodimeric archaeoeukaryotic primases and primes sequence-specifically.Nucleic Acids Res. 2023 Jun 9;51(10):5087-5105. doi: 10.1093/nar/gkad261. Nucleic Acids Res. 2023. PMID: 37099378 Free PMC article.
References
-
- Pellegrini L. (2012) The Pol α-primase complex. Subcell. Biochem. 62, 157–169 - PubMed
Publication types
MeSH terms
Substances
Associated data
- Actions
Grants and funding
LinkOut - more resources
Full Text Sources
Molecular Biology Databases