Bacterial Sigma Factors and Anti-Sigma Factors: Structure, Function and Distribution
- PMID: 26131973
- PMCID: PMC4598750
- DOI: 10.3390/biom5031245
Bacterial Sigma Factors and Anti-Sigma Factors: Structure, Function and Distribution
Abstract
Sigma factors are multi-domain subunits of bacterial RNA polymerase (RNAP) that play critical roles in transcription initiation, including the recognition and opening of promoters as well as the initial steps in RNA synthesis. This review focuses on the structure and function of the major sigma-70 class that includes the housekeeping sigma factor (Group 1) that directs the bulk of transcription during active growth, and structurally-related alternative sigma factors (Groups 2-4) that control a wide variety of adaptive responses such as morphological development and the management of stress. A recurring theme in sigma factor control is their sequestration by anti-sigma factors that occlude their RNAP-binding determinants. Sigma factors are then released through a wide variety of mechanisms, often involving branched signal transduction pathways that allow the integration of distinct signals. Three major strategies for sigma release are discussed: regulated proteolysis, partner-switching, and direct sensing by the anti-sigma factor.
Keywords: RIP; RNA polymerase; anti-sigma; extracytoplasmic; partner-switching; sigma; signal transduction; stress; transcription.
Figures
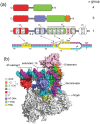
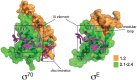
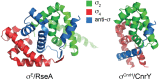
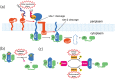
Similar articles
-
Recent advances in the characterization of Crl, the unconventional activator of the stress sigma factor σS/RpoS.Biomol Concepts. 2016 Jun 1;7(3):197-204. doi: 10.1515/bmc-2016-0006. Biomol Concepts. 2016. PMID: 27180360 Review.
-
Extra Cytoplasmic Function sigma factors, recent structural insights into promoter recognition and regulation.Curr Opin Struct Biol. 2015 Feb;30:71-78. doi: 10.1016/j.sbi.2015.01.006. Epub 2015 Feb 9. Curr Opin Struct Biol. 2015. PMID: 25678040 Review.
-
The σ enigma: bacterial σ factors, archaeal TFB and eukaryotic TFIIB are homologs.Transcription. 2014;5(4):e967599. doi: 10.4161/21541264.2014.967599. Transcription. 2014. PMID: 25483602 Free PMC article.
-
Structural basis for sigma factor mimicry in the general stress response of Alphaproteobacteria.Proc Natl Acad Sci U S A. 2012 May 22;109(21):E1405-14. doi: 10.1073/pnas.1117003109. Epub 2012 May 1. Proc Natl Acad Sci U S A. 2012. PMID: 22550171 Free PMC article.
-
Bacterial sigma factors: a historical, structural, and genomic perspective.Annu Rev Microbiol. 2014;68:357-76. doi: 10.1146/annurev-micro-092412-155737. Epub 2014 Jun 18. Annu Rev Microbiol. 2014. PMID: 25002089 Review.
Cited by
-
An Extracytoplasmic Function Sigma/Anti-Sigma Factor System Regulates β-Glucanase Expression in Tannerella forsythia in Response to Fusobacterium nucleatum Sensing.J Bacteriol. 2022 Dec 20;204(12):e0031322. doi: 10.1128/jb.00313-22. Epub 2022 Nov 30. J Bacteriol. 2022. PMID: 36448787 Free PMC article.
-
Bacterial nanotubes as a manifestation of cell death.Nat Commun. 2020 Oct 2;11(1):4963. doi: 10.1038/s41467-020-18800-2. Nat Commun. 2020. PMID: 33009406 Free PMC article.
-
Modulating Gene Expression within a Microbiome Based on Computational Models.Biology (Basel). 2022 Aug 31;11(9):1301. doi: 10.3390/biology11091301. Biology (Basel). 2022. PMID: 36138780 Free PMC article.
-
The Stringent Stress Response Controls Proteases and Global Regulators under Optimal Growth Conditions in Pseudomonas aeruginosa.mSystems. 2020 Aug 4;5(4):e00495-20. doi: 10.1128/mSystems.00495-20. mSystems. 2020. PMID: 32753509 Free PMC article.
-
β-Lactam Resistance in Azospirillum baldaniorum Sp245 Is Mediated by Lytic Transglycosylase and β-Lactamase and Regulated by a Cascade of RpoE7→RpoH3 Sigma Factors.J Bacteriol. 2022 Apr 19;204(4):e0001022. doi: 10.1128/jb.00010-22. Epub 2022 Mar 30. J Bacteriol. 2022. PMID: 35352964 Free PMC article.
References
Publication types
MeSH terms
Substances
Grants and funding
LinkOut - more resources
Full Text Sources
Other Literature Sources
Miscellaneous