Mechanosensory hairs in bumblebees (Bombus terrestris) detect weak electric fields
- PMID: 27247399
- PMCID: PMC4932954
- DOI: 10.1073/pnas.1601624113
Mechanosensory hairs in bumblebees (Bombus terrestris) detect weak electric fields
Abstract
Bumblebees (Bombus terrestris) use information from surrounding electric fields to make foraging decisions. Electroreception in air, a nonconductive medium, is a recently discovered sensory capacity of insects, yet the sensory mechanisms remain elusive. Here, we investigate two putative electric field sensors: antennae and mechanosensory hairs. Examining their mechanical and neural response, we show that electric fields cause deflections in both antennae and hairs. Hairs respond with a greater median velocity, displacement, and angular displacement than antennae. Extracellular recordings from the antennae do not show any electrophysiological correlates to these mechanical deflections. In contrast, hair deflections in response to an electric field elicited neural activity. Mechanical deflections of both hairs and antennae increase with the electric charge carried by the bumblebee. From this evidence, we conclude that sensory hairs are a site of electroreception in the bumblebee.
Keywords: bees; behavior; electric fields; sensory biology.
Conflict of interest statement
The authors declare no conflict of interest.
Figures
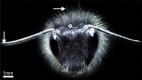
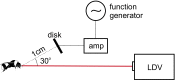
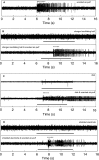
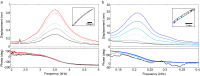
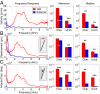
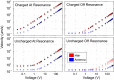
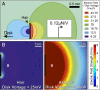
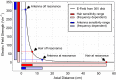
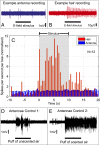
Comment in
-
Electric fields of flowers stimulate the sensory hairs of bumble bees.Proc Natl Acad Sci U S A. 2016 Jun 28;113(26):7020-1. doi: 10.1073/pnas.1607426113. Epub 2016 Jun 20. Proc Natl Acad Sci U S A. 2016. PMID: 27325771 Free PMC article. No abstract available.
Similar articles
-
Bumblebee hairs as electric and air motion sensors: theoretical analysis of an isolated hair.J R Soc Interface. 2020 Jul;17(168):20200146. doi: 10.1098/rsif.2020.0146. Epub 2020 Jul 8. J R Soc Interface. 2020. PMID: 32634368 Free PMC article.
-
The final moments of landing in bumblebees, Bombus terrestris.J Comp Physiol A Neuroethol Sens Neural Behav Physiol. 2016 Apr;202(4):277-85. doi: 10.1007/s00359-016-1073-4. Epub 2016 Feb 11. J Comp Physiol A Neuroethol Sens Neural Behav Physiol. 2016. PMID: 26868924
-
Passive electrolocation in terrestrial arthropods: Theoretical modelling of location detection.J Theor Biol. 2023 Feb 7;558:111357. doi: 10.1016/j.jtbi.2022.111357. Epub 2022 Nov 21. J Theor Biol. 2023. PMID: 36410450 Free PMC article.
-
Aerial electroreception.Curr Biol. 2024 Oct 21;34(20):R1018-R1023. doi: 10.1016/j.cub.2024.06.028. Curr Biol. 2024. PMID: 39437720
-
Antennal hearing in insects--new findings, new questions.Hear Res. 2011 Mar;273(1-2):7-13. doi: 10.1016/j.heares.2010.03.092. Epub 2010 Apr 27. Hear Res. 2011. PMID: 20430076 Review.
Cited by
-
Repelling Aedes aegypti mosquitoes with electric fields using insulated conductor wires.PLoS Negl Trop Dis. 2024 Sep 13;18(9):e0012493. doi: 10.1371/journal.pntd.0012493. eCollection 2024 Sep. PLoS Negl Trop Dis. 2024. PMID: 39269948 Free PMC article.
-
An analysis of time-varying dynamics in electrically sensitive arthropod hairs to understand real-world electrical sensing.J R Soc Interface. 2023 Aug;20(205):20230177. doi: 10.1098/rsif.2023.0177. Epub 2023 Aug 9. J R Soc Interface. 2023. PMID: 37553992 Free PMC article.
-
Bumblebee electric charge stimulates floral volatile emissions in Petunia integrifolia but not in Antirrhinum majus.Naturwissenschaften. 2021 Sep 14;108(5):44. doi: 10.1007/s00114-021-01740-2. Naturwissenschaften. 2021. PMID: 34519874 Free PMC article.
-
Interactions between electromagnetic radiation and biological systems.iScience. 2024 Feb 10;27(3):109201. doi: 10.1016/j.isci.2024.109201. eCollection 2024 Mar 15. iScience. 2024. PMID: 38433903 Free PMC article. Review.
-
The bee, the flower, and the electric field: electric ecology and aerial electroreception.J Comp Physiol A Neuroethol Sens Neural Behav Physiol. 2017 Sep;203(9):737-748. doi: 10.1007/s00359-017-1176-6. Epub 2017 Jun 24. J Comp Physiol A Neuroethol Sens Neural Behav Physiol. 2017. PMID: 28647753 Free PMC article. Review.
References
-
- Kalmijn AJ. The electric sense of sharks and rays. J Exp Biol. 1971;55(2):371–383. - PubMed
-
- Murray RW. The response of the ampullae of Lorenzini of elasmobranchs to electrical stimulation. J Exp Biol. 1962;39:119–128. - PubMed
-
- Fritzsch B, Wake MH. Electro-reception in amphibians. Am Sci. 1984;72(3):228.
-
- Tong SL, Bullock TH. Electroreceptive representation and its dynamics in the cerebellum of the catfish, Ictalarusnebulosus (Ictaluridae, Siluriformes) J Comput Phys. 1982;145:289–298.
-
- Bullock TH, Northcutt RG. A new electroreceptive teleost – Xenomystusnigri (Osteoglossiformes notopteridae) J Comp Physiol. 1982;3:345–352.
Publication types
MeSH terms
LinkOut - more resources
Full Text Sources
Other Literature Sources