Magma reservoir dynamics at Toba caldera, Indonesia, recorded by oxygen isotope zoning in quartz
- PMID: 28120860
- PMCID: PMC5264179
- DOI: 10.1038/srep40624
Magma reservoir dynamics at Toba caldera, Indonesia, recorded by oxygen isotope zoning in quartz
Abstract
Quartz is a common phase in high-silica igneous rocks and is resistant to post-eruptive alteration, thus offering a reliable record of magmatic processes in silicic magma systems. Here we employ the 75 ka Toba super-eruption as a case study to show that quartz can resolve late-stage temporal changes in magmatic δ18O values. Overall, Toba quartz crystals exhibit comparatively high δ18O values, up to 10.2‰, due to magma residence within, and assimilation of, local granite basement. However, some 40% of the analysed quartz crystals display a decrease in δ18O values in outermost growth zones compared to their cores, with values as low as 6.7‰ (maximum ∆core-rim = 1.8‰). These lower values are consistent with the limited zircon record available for Toba, and the crystallisation history of Toba quartz traces an influx of a low-δ18O component into the magma reservoir just prior to eruption. Here we argue that this late-stage low-δ18O component is derived from hydrothermally-altered roof material. Our study demonstrates that quartz isotope stratigraphy can resolve magmatic events that may remain undetected by whole-rock or zircon isotope studies, and that assimilation of altered roof material may represent a viable eruption trigger in large Toba-style magmatic systems.
Figures
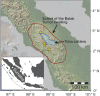
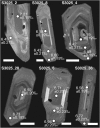
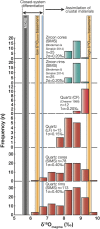
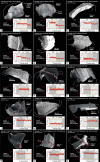
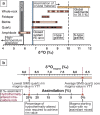
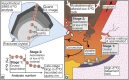
Similar articles
-
Magmatic history of the Oldest Toba Tuff inferred from zircon U-Pb geochronology.Sci Rep. 2020 Oct 15;10(1):17506. doi: 10.1038/s41598-020-74512-z. Sci Rep. 2020. PMID: 33060763 Free PMC article.
-
Growth and thermal maturation of the Toba magma reservoir.Proc Natl Acad Sci U S A. 2021 Nov 9;118(45):e2101695118. doi: 10.1073/pnas.2101695118. Proc Natl Acad Sci U S A. 2021. PMID: 34725149 Free PMC article.
-
Post-supereruption recovery at Toba Caldera.Nat Commun. 2017 May 16;8:15248. doi: 10.1038/ncomms15248. Nat Commun. 2017. PMID: 28508876 Free PMC article.
-
Magmatic-hydrothermal fluid evolution of the tin-polymetallic metallogenic systems from the Weilasituo ore district, Northeast China.Sci Rep. 2024 Feb 6;14(1):3006. doi: 10.1038/s41598-024-53579-y. Sci Rep. 2024. PMID: 38321094 Free PMC article.
-
The carbon isotope composition of ancient CO2 based on higher-plant organic matter.Philos Trans A Math Phys Eng Sci. 2002 Apr 15;360(1793):633-58. doi: 10.1098/rsta.2001.0965. Philos Trans A Math Phys Eng Sci. 2002. PMID: 12804297 Review.
Cited by
-
Derivation of intermediate to silicic magma from the basalt analyzed at the Vega 2 landing site, Venus.PLoS One. 2018 Mar 27;13(3):e0194155. doi: 10.1371/journal.pone.0194155. eCollection 2018. PLoS One. 2018. PMID: 29584745 Free PMC article.
-
Magmatic history of the Oldest Toba Tuff inferred from zircon U-Pb geochronology.Sci Rep. 2020 Oct 15;10(1):17506. doi: 10.1038/s41598-020-74512-z. Sci Rep. 2020. PMID: 33060763 Free PMC article.
-
Sunda arc mantle source δ18O value revealed by intracrystal isotope analysis.Nat Commun. 2021 Jun 24;12(1):3930. doi: 10.1038/s41467-021-24143-3. Nat Commun. 2021. PMID: 34168147 Free PMC article.
References
-
- Davidson J. P., Hora J. M., Garrison J. M. & Dungan M. A. Crustal forensics in arc magmas. J. Volcanol. Geotherm. Res. 140, 157–170 (2005).
-
- Valley J. W. & Kita N. T. In situ oxygen isotope geochemistry by ion microprobe. Mineral. Assoc. Canada Short Course 19–63 (2009).
-
- Valley J. W. et al.. Nano- and micro-geochronology in Hadean and Archean zircons by atom-probe tomography and SIMS: New tools for old minerals. Am. Mineral. 100 (2015).
-
- Valley J. W. Oxygen Isotopes in Zircon. Rev. Mineral. Geochemistry 53, 343–385 (2003).
-
- Ferry J. M., Kitajima K., Strickland A. & Valley J. W. Ion microprobe survey of the grain-scale oxygen isotope geochemistry of minerals in metamorphic rocks. Geochim. Cosmochim. Acta 144, 403–433 (2014).
Publication types
LinkOut - more resources
Full Text Sources
Other Literature Sources