Long-term mutual phase locking of picosecond pulse pairs generated by a semiconductor nanowire laser
- PMID: 28534489
- PMCID: PMC5457509
- DOI: 10.1038/ncomms15521
Long-term mutual phase locking of picosecond pulse pairs generated by a semiconductor nanowire laser
Abstract
The ability to generate phase-stabilized trains of ultrafast laser pulses by mode-locking underpins photonics research in fields, such as precision metrology and spectroscopy. However, the complexity of conventional mode-locked laser systems has hindered their realization at the nanoscale. Here we demonstrate that GaAs-AlGaAs nanowire lasers are capable of emitting pairs of phase-locked picosecond laser pulses with a repetition frequency up to 200 GHz when subject to incoherent pulsed optical excitation. By probing the two-pulse interference spectra, we show that pulse pairs remain mutually coherent over timescales extending to 30 ps, much longer than the emitted laser pulse duration (≤3 ps). Simulations performed by solving the optical Bloch equations produce good quantitative agreement with experiments, revealing how the phase information is stored in the gain medium close to transparency. Our results open the way to phase locking of nanowires integrated onto photonic circuits, optical injection locking and applications, such as on-chip Ramsey comb spectroscopy.
Conflict of interest statement
The authors declare no competing financial interests.
Figures
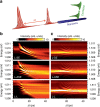
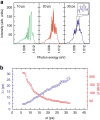
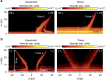
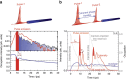
Similar articles
-
Passively mode-locked III-V/silicon laser with continuous-wave optical injection.Opt Express. 2015 Mar 9;23(5):6392-9. doi: 10.1364/OE.23.006392. Opt Express. 2015. PMID: 25836859
-
1.8-THz-wide optical frequency comb emitted from monolithic passively mode-locked semiconductor quantum-well laser.Opt Lett. 2017 Oct 1;42(19):3872-3875. doi: 10.1364/OL.42.003872. Opt Lett. 2017. PMID: 28957148
-
Ultrashort pulse generation by semiconductor mode-locked lasers at 760 nm.Opt Express. 2014 Oct 20;22(21):25940-6. doi: 10.1364/OE.22.025940. Opt Express. 2014. PMID: 25401627
-
Single-Mode Semiconductor Nanowire Lasers With Coupled Cavities.Front Chem. 2021 Jan 15;8:631870. doi: 10.3389/fchem.2020.631870. eCollection 2020. Front Chem. 2021. PMID: 33520944 Free PMC article. Review.
-
Effects of pulse shaping in laser spectroscopy and nuclear magnetic resonance.Science. 1988 Nov 11;242(4880):878-84. doi: 10.1126/science.3055299. Science. 1988. PMID: 3055299 Review.
Cited by
-
Observation of polarity-switchable photoconductivity in III-nitride/MoSx core-shell nanowires.Light Sci Appl. 2022 Jul 19;11(1):227. doi: 10.1038/s41377-022-00912-7. Light Sci Appl. 2022. PMID: 35853856 Free PMC article.
-
Pulse-doubling perovskite nanowire lasers enabled by phonon-assisted multistep energy funneling.Light Sci Appl. 2024 Jul 17;13(1):170. doi: 10.1038/s41377-024-01494-2. Light Sci Appl. 2024. PMID: 39019895 Free PMC article.
-
High-Performance Multiwavelength GaNAs Single Nanowire Lasers.ACS Nano. 2024 Jan 16;18(2):1477-1484. doi: 10.1021/acsnano.3c07980. Epub 2024 Jan 2. ACS Nano. 2024. PMID: 38166147 Free PMC article.
References
-
- Del'Haye P. et al.. Optical frequency comb generation from a monolithic microresonator. Nature 450, 1214–1217 (2007). - PubMed
-
- Nakayama Y. et al.. Tunable nanowire nonlinear optical probe. Nature 447, 1098–1101 (2007). - PubMed
-
- Piccione B., Cho C., van Vugt L. K. & Agarwal R. All-optical active switching in individual semiconductor nanowires. Nat. Nanotechnol. 7, 640–645 (2012). - PubMed
-
- Yan R., Gargas D. & Yang P. Nanowire photonics. Nat. Photon. 3, 569–576 (2009).
Publication types
LinkOut - more resources
Full Text Sources
Other Literature Sources