Evolution and Developmental Diversity of Skin Spines in Pufferfishes
- PMID: 31353167
- PMCID: PMC6831732
- DOI: 10.1016/j.isci.2019.06.003
Evolution and Developmental Diversity of Skin Spines in Pufferfishes
Abstract
Teleost fishes develop remarkable varieties of skin ornaments. The developmental basis of these structures is poorly understood. The order Tetraodontiformes includes diverse fishes such as the ocean sunfishes, triggerfishes, and pufferfishes, which exhibit a vast assortment of scale derivatives. Pufferfishes possess some of the most extreme scale derivatives, dermal spines, erected during their characteristic puffing behavior. We demonstrate that pufferfish scale-less spines develop through conserved gene interactions that underlie general vertebrate skin appendage formation, including feathers and hair. Spine development retains conservation of the EDA (ectodysplasin) signaling pathway, important for the development of diverse vertebrate skin appendages, including these modified scale-less spines of pufferfish. Further modification of genetic signaling from both CRISPR-Cas9 and small molecule inhibition leads to loss or reduction of spine coverage, providing a mechanism for skin appendage diversification observed throughout the pufferfishes. Pufferfish spines have evolved broad variations in body coverage, enabling adaptation to diverse ecological niches.
Keywords: Biological Sciences; Evolutionary Biology; Evolutionary Developmental Biology.
Copyright © 2019 The Author(s). Published by Elsevier Inc. All rights reserved.
Conflict of interest statement
The authors declare no competing interests.
Figures
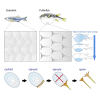
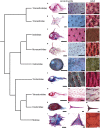
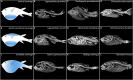
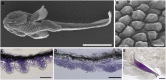
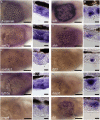
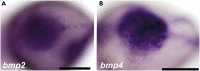
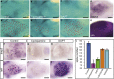
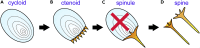
Similar articles
-
Replacing the first-generation dentition in pufferfish with a unique beak.Proc Natl Acad Sci U S A. 2012 May 22;109(21):8179-84. doi: 10.1073/pnas.1119635109. Epub 2012 May 7. Proc Natl Acad Sci U S A. 2012. PMID: 22566613 Free PMC article.
-
Do habitat shifts drive diversification in teleost fishes? An example from the pufferfishes (Tetraodontidae).J Evol Biol. 2013 May;26(5):1003-18. doi: 10.1111/jeb.12112. Epub 2013 Mar 15. J Evol Biol. 2013. PMID: 23496826
-
Spatially restricted dental regeneration drives pufferfish beak development.Proc Natl Acad Sci U S A. 2017 May 30;114(22):E4425-E4434. doi: 10.1073/pnas.1702909114. Epub 2017 May 15. Proc Natl Acad Sci U S A. 2017. PMID: 28507130 Free PMC article.
-
[The problem of the skin derivatives' origin in the amniote evolution. The skin appendages--scale, feather, and hair].Zh Obshch Biol. 2008 Mar-Apr;69(2):130-51. Zh Obshch Biol. 2008. PMID: 18669304 Review. Russian.
-
Avian skin development and the evolutionary origin of feathers.J Exp Zool B Mol Dev Evol. 2003 Aug 15;298(1):57-72. doi: 10.1002/jez.b.26. J Exp Zool B Mol Dev Evol. 2003. PMID: 12949769 Review.
Cited by
-
Leafy and weedy seadragon genomes connect genic and repetitive DNA features to the extravagant biology of syngnathid fishes.Proc Natl Acad Sci U S A. 2022 Jun 28;119(26):e2119602119. doi: 10.1073/pnas.2119602119. Epub 2022 Jun 22. Proc Natl Acad Sci U S A. 2022. PMID: 35733255 Free PMC article.
-
The ectodysplasin-A receptor is a candidate gene for lateral plate number variation in stickleback fish.G3 (Bethesda). 2022 May 30;12(6):jkac077. doi: 10.1093/g3journal/jkac077. G3 (Bethesda). 2022. PMID: 35377433 Free PMC article.
-
Expression variations in ectodysplasin-A gene (eda) may contribute to morphological divergence of scales in haplochromine cichlids.BMC Ecol Evol. 2022 Mar 10;22(1):28. doi: 10.1186/s12862-022-01984-0. BMC Ecol Evol. 2022. PMID: 35272610 Free PMC article.
-
The Exploitation of Toxic Fish from the Terminal Pleistocene in Maritime Southeast Asia: A Case Study from the Mindoro Archaeological Sites, Philippines.Animals (Basel). 2023 Jun 26;13(13):2113. doi: 10.3390/ani13132113. Animals (Basel). 2023. PMID: 37443911 Free PMC article.
-
The Genetic Basis of Scale-Loss Phenotype in the Rapid Radiation of Takifugu Fishes.Genes (Basel). 2019 Dec 10;10(12):1027. doi: 10.3390/genes10121027. Genes (Basel). 2019. PMID: 31835491 Free PMC article.
References
-
- Bauer H., Meier A., Hild M., Stachel S., Economides A., Hazelett D., Harland R.M., Hammerschmidt M. Follistatin and Noggin are excluded from the zebrafish organizer. Dev. Biol. 1998;204:488–507. - PubMed
-
- Biggs L.C., Mikkola M.L. Early inductive events in ectodermal appendage morphogenesis. Semin. Cell Dev. Biol. 2014;25–26:11–21. - PubMed
LinkOut - more resources
Full Text Sources