Trophodynamics of Southern Ocean pteropods on the southern Kerguelen Plateau
- PMID: 31380076
- PMCID: PMC6662407
- DOI: 10.1002/ece3.5380
Trophodynamics of Southern Ocean pteropods on the southern Kerguelen Plateau
Abstract
Pteropods are a group of small marine gastropods that are highly sensitive to multiple stressors associated with climate change. Their trophic ecology is not well studied, with most research having focused primarily on the effects of ocean acidification on their fragile, aragonite shells. Stable isotopes analysis coupled with isotope-based Bayesian niche metrics is useful for characterizing the trophic structure of biological assemblages. These approaches have not been implemented for pteropod assemblages. We used isotope-based Bayesian niche metrics to investigate the trophic relationships of three co-occurring pteropod species, with distinct feeding behaviors, sampled from the Southern Kerguelen Plateau area in the Indian Sector of the Southern Ocean-a biologically and economically important but poorly studied region. Two of these species were gymnosomes (shell-less pteropods), which are traditionally regarded as specialist predators on other pteropods, and the third species was a thecosome (shelled pteropod), which are typically generalist omnivores. For each species, we aimed to understand (a) variability and overlap among isotopic niches; and (b) whether there was a relationship between body size and trophic position. Observed isotopic niche areas were broadest for gymnosomes, especially Clione limacina antarctica, whose observed isotopic niche area was wider than expected on both δ13C and δ15N value axes. We also found that trophic position significantly increased with increasing body length for Spongiobranchaea australis. We found no indication of a dietary shift toward increased trophic position with increasing body size for Clio pyramidata f. sulcata. Trophic positions ranged from 2.8 to 3.5, revealing an assemblage composed of both primary and secondary consumer behaviors. This study provides a comprehensive comparative analysis on trophodynamics in Southern Ocean pteropod species, and supports previous studies using gut content, fatty acid and stable isotope analyses. Combined, our results illustrate differences in intraspecific trophic behavior that may be attributed to differential feeding strategies at species level.
Keywords: Clio pyramidata; Clione limacina; Spongiobranchaea australis; isotopic niche; size‐based; trophic position.
Conflict of interest statement
None declared.
Figures
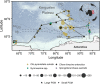
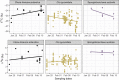
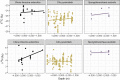
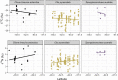
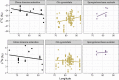
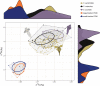
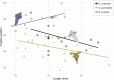
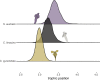
Similar articles
-
Can lipid removal affect interpretation of resource partitioning from stable isotopes in Southern Ocean pteropods?Rapid Commun Mass Spectrom. 2019 Mar 30;33(6):569-578. doi: 10.1002/rcm.8384. Rapid Commun Mass Spectrom. 2019. PMID: 30635929
-
Lipids and buoyancy in Southern ocean pteropods.Lipids. 1997 Oct;32(10):1093-100. doi: 10.1007/s11745-997-0141-x. Lipids. 1997. PMID: 9358436
-
Combined stress of ocean acidification and warming influence survival and drives differential gene expression patterns in the Antarctic pteropod, Limacina helicina antarctica.Conserv Physiol. 2020 Mar 26;8(1):coaa013. doi: 10.1093/conphys/coaa013. eCollection 2020. Conserv Physiol. 2020. PMID: 32257214 Free PMC article.
-
Improving stable isotope assessments of inter- and intra-species variation in coral reef fish trophic strategies.Ecol Evol. 2022 Sep 13;12(9):e9221. doi: 10.1002/ece3.9221. eCollection 2022 Sep. Ecol Evol. 2022. PMID: 36172294 Free PMC article.
-
Evolution and biomineralization of pteropod shells.J Struct Biol. 2021 Dec;213(4):107779. doi: 10.1016/j.jsb.2021.107779. Epub 2021 Aug 30. J Struct Biol. 2021. PMID: 34474158 Review.
References
-
- Agostini, S. , Harvey, B. P. , Wada, S. , Kon, K. , Milazzo, M. , Inaba, K. , & Hall‐Spencer, J. M. (2018). Ocean acidification drives community shifts towards simplified non‐calcified habitats in a subtropical−temperate transition zone. Scientific Reports, 8, 5–10. 10.1038/s41598-018-29251-7 - DOI - PMC - PubMed
-
- Bas, M. , Briz i Godino, I. , Álvarez, M. , Vales, D. G. , Crespo, E. A. , & Cardona, L. (2019). Back to the future? Late Holocene marine food web structure in a warm climatic phase as a predictor of trophodynamics in a warmer South‐Western Atlantic Ocean. Global Change Biology, 25, 404–419. 10.1111/gcb.14523 - DOI - PubMed
-
- Bearhop, S. , Adams, C. E. , Waldron, S. , Fuller, R. A. , & Macleod, H. (2004). Determining trophic niche width: A novel approach using stable isotope analysis. Journal of Animal Ecology, 73, 1007–1012. 10.1111/j.0021-8790.2004.00861.x - DOI
-
- Bednaršek, N. , Harvey, C. J. , Kaplan, I. C. , Feely, R. A. , & Možina, J. (2016). Pteropods on the edge: Cumulative effects of ocean acidification, warming, and deoxygenation. Progress in Oceanography, 145, 1–24. 10.1016/j.pocean.2016.04.002 - DOI
LinkOut - more resources
Full Text Sources