Genes lost during the transition from land to water in cetaceans highlight genomic changes associated with aquatic adaptations
- PMID: 31579821
- PMCID: PMC6760925
- DOI: 10.1126/sciadv.aaw6671
Genes lost during the transition from land to water in cetaceans highlight genomic changes associated with aquatic adaptations
Abstract
The transition from land to water in whales and dolphins (cetaceans) was accompanied by remarkable adaptations. To reveal genomic changes that occurred during this transition, we screened for protein-coding genes that were inactivated in the ancestral cetacean lineage. We found 85 gene losses. Some of these were likely beneficial for cetaceans, for example, by reducing the risk of thrombus formation during diving (F12 and KLKB1), erroneous DNA damage repair (POLM), and oxidative stress-induced lung inflammation (MAP3K19). Additional gene losses may reflect other diving-related adaptations, such as enhanced vasoconstriction during the diving response (mediated by SLC6A18) and altered pulmonary surfactant composition (SEC14L3), while loss of SLC4A9 relates to a reduced need for saliva. Last, loss of melatonin synthesis and receptor genes (AANAT, ASMT, and MTNR1A/B) may have been a precondition for adopting unihemispheric sleep. Our findings suggest that some genes lost in ancestral cetaceans were likely involved in adapting to a fully aquatic lifestyle.
Copyright © 2019 The Authors, some rights reserved; exclusive licensee American Association for the Advancement of Science. No claim to original U.S. Government Works. Distributed under a Creative Commons Attribution NonCommercial License 4.0 (CC BY-NC).
Figures
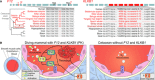
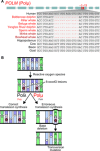
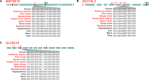
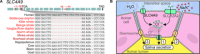
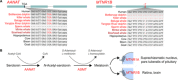
Similar articles
-
Genomic and anatomical comparisons of skin support independent adaptation to life in water by cetaceans and hippos.Curr Biol. 2021 May 24;31(10):2124-2139.e3. doi: 10.1016/j.cub.2021.02.057. Epub 2021 Apr 1. Curr Biol. 2021. PMID: 33798433 Free PMC article.
-
Molecular evolution tracks macroevolutionary transitions in Cetacea.Trends Ecol Evol. 2014 Jun;29(6):336-46. doi: 10.1016/j.tree.2014.04.001. Epub 2014 May 1. Trends Ecol Evol. 2014. PMID: 24794916 Review.
-
Adaptive evolution and functional constraint at TLR4 during the secondary aquatic adaptation and diversification of cetaceans.BMC Evol Biol. 2012 Mar 24;12:39. doi: 10.1186/1471-2148-12-39. BMC Evol Biol. 2012. PMID: 22443485 Free PMC article.
-
Positive Selection and Inactivation in the Vision and Hearing Genes of Cetaceans.Mol Biol Evol. 2020 Jul 1;37(7):2069-2083. doi: 10.1093/molbev/msaa070. Mol Biol Evol. 2020. PMID: 32170943
-
An evolutionary history of F12 gene: Emergence, loss, and vulnerability with the environment as a driver.Bioessays. 2023 Dec;45(12):e2300077. doi: 10.1002/bies.202300077. Epub 2023 Sep 26. Bioessays. 2023. PMID: 37750435 Review.
Cited by
-
Phylogeny, Ecology, and Gene Families Covariation Shaped the Olfactory Subgenome of Rodents.Genome Biol Evol. 2023 Nov 1;15(11):evad197. doi: 10.1093/gbe/evad197. Genome Biol Evol. 2023. PMID: 37972291 Free PMC article.
-
Adaptive Evolution of the OAS Gene Family Provides New Insights into the Antiviral Ability of Laurasiatherian Mammals.Animals (Basel). 2023 Jan 6;13(2):209. doi: 10.3390/ani13020209. Animals (Basel). 2023. PMID: 36670749 Free PMC article.
-
Genomic and anatomical comparisons of skin support independent adaptation to life in water by cetaceans and hippos.Curr Biol. 2021 May 24;31(10):2124-2139.e3. doi: 10.1016/j.cub.2021.02.057. Epub 2021 Apr 1. Curr Biol. 2021. PMID: 33798433 Free PMC article.
-
A genome alignment of 120 mammals highlights ultraconserved element variability and placenta-associated enhancers.Gigascience. 2020 Jan 1;9(1):giz159. doi: 10.1093/gigascience/giz159. Gigascience. 2020. PMID: 31899510 Free PMC article.
-
Parallel Independent Losses of G-Type Lysozyme Genes in Hairless Aquatic Mammals.Genome Biol Evol. 2021 Sep 1;13(9):evab201. doi: 10.1093/gbe/evab201. Genome Biol Evol. 2021. PMID: 34450623 Free PMC article.
References
-
- Thewissen J. G. M., Cooper L. N., Clementz M. T., Bajpai S., Tiwari B. N., Whales originated from aquatic artiodactyls in the Eocene epoch of India. Nature 450, 1190–1194 (2007). - PubMed
-
- Gatesy J., Geisler J. H., Chang J., Buell C., Berta A., Meredith R. W., Springer M. S., McGowen M. R., A phylogenetic blueprint for a modern whale. Mol. Phylogenet. Evol. 66, 479–506 (2013). - PubMed
-
- A. Berta, J. L. Sumich, K. M. Kovacs, Marine Mammals: Evolutionary Biology (Academic Press, 2005).
-
- Kooyman G. L., Ponganis P. J., The physiological basis of diving to depth: Birds and mammals. Annu. Rev. Physiol. 60, 19–32 (1998). - PubMed
-
- McGowen M. R., Gatesy J., Wildman D. E., Molecular evolution tracks macroevolutionary transitions in Cetacea. Trends Ecol. Evol. 29, 336–346 (2014). - PubMed
Publication types
MeSH terms
Associated data
LinkOut - more resources
Full Text Sources
Other Literature Sources
Miscellaneous