Evaporation coefficient and condensation coefficient of vapor under high gas pressure conditions
- PMID: 32424295
- PMCID: PMC7235219
- DOI: 10.1038/s41598-020-64905-5
Evaporation coefficient and condensation coefficient of vapor under high gas pressure conditions
Erratum in
-
Author Correction: Evaporation coefficient and condensation coefficient of vapor under high gas pressure conditions.Sci Rep. 2020 Jul 1;10(1):11094. doi: 10.1038/s41598-020-68283-w. Sci Rep. 2020. PMID: 32606333 Free PMC article.
Abstract
We investigated the evaporation and condensation coefficients of vapor, which represent evaporation and condensation rates of vapor molecules, under high gas pressure (high gas density) conditions in a system of a vapor/gas-liquid equilibrium state. The mixture gas is composed of condensable gas (vapor) and non-condensable gas (NC gas) molecules. We performed numerical simulations of vapor/gas-liquid equilibrium systems with the Enskog-Vlasov direct simulation Monte Carlo (EVDSMC) method. As a result of the simulations, we found that the evaporation and condensation fluxes decrease with increasing NC gas pressure, which leads to a decrease in the evaporation and condensation coefficients of vapor molecules. Especially, under extremely high gas pressure conditions, the values of these coefficients are close to zero, which means the vapor molecules cannot evaporate and condensate at the interface. Moreover, we found that the vapor molecules behave as NC gas molecules under high gas pressure conditions. We also discussed the reason why NC gas molecules interfere with evaporation and condensation of vapor molecules at the vapor/gas-liquid interface.
Conflict of interest statement
The authors declare no competing interests.
Figures
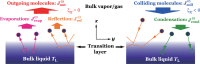
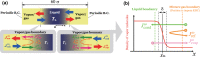
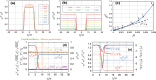
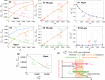
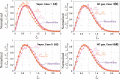
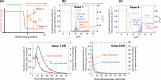
Similar articles
-
Molecular dynamics study on characteristics of reflection and condensation molecules at vapor-liquid equilibrium state.PLoS One. 2021 Mar 16;16(3):e0248660. doi: 10.1371/journal.pone.0248660. eCollection 2021. PLoS One. 2021. PMID: 33725026 Free PMC article.
-
Molecular dynamics study on condensation/evaporation coefficients of chain molecules at liquid-vapor interface.J Chem Phys. 2015 Jul 7;143(1):014706. doi: 10.1063/1.4923261. J Chem Phys. 2015. PMID: 26156491
-
Evaporation of Lennard-Jones fluids.J Chem Phys. 2011 Jun 14;134(22):224704. doi: 10.1063/1.3595260. J Chem Phys. 2011. PMID: 21682530
-
Thermophysical Properties of the Lennard-Jones Fluid: Database and Data Assessment.J Chem Inf Model. 2019 Oct 28;59(10):4248-4265. doi: 10.1021/acs.jcim.9b00620. Epub 2019 Oct 14. J Chem Inf Model. 2019. PMID: 31609113 Review.
-
Binary Solvent Swap Processing in a Bubble Column in Batch and Continuous Modes.Org Process Res Dev. 2022 Apr 15;26(4):1191-1201. doi: 10.1021/acs.oprd.1c00455. Epub 2022 Mar 16. Org Process Res Dev. 2022. PMID: 35464823 Free PMC article. Review.
Cited by
-
Molecular dynamics study on characteristics of reflection and condensation molecules at vapor-liquid equilibrium state.PLoS One. 2021 Mar 16;16(3):e0248660. doi: 10.1371/journal.pone.0248660. eCollection 2021. PLoS One. 2021. PMID: 33725026 Free PMC article.
-
Effect of Low Environmental Pressure on Sintering Behavior of NASICON-Type Li1.3Al0.3Ti1.7(PO4)3 Solid Electrolytes: An In Situ ESEM Study.Cryst Growth Des. 2023 Feb 17;23(3):1522-1529. doi: 10.1021/acs.cgd.2c01098. eCollection 2023 Mar 1. Cryst Growth Des. 2023. PMID: 36879774 Free PMC article.
References
-
- Langmuir I. Evaporation, condensation, and adsorption. Nature. 1932;130:515–516.
-
- Al-Shammiri M, Safar M. Multi-effect distillation plants: state of the art. Desalination. 1999;126:45–59. doi: 10.1016/S0011-9164(99)00154-X. - DOI
-
- Sone, Y. Molecular Gas Dynamics (Birkhäuser, 2007).
LinkOut - more resources
Full Text Sources
Research Materials