Group I PAKs in myelin formation and repair of the central nervous system: what, when, and how
- PMID: 34811887
- PMCID: PMC8917091
- DOI: 10.1111/brv.12815
Group I PAKs in myelin formation and repair of the central nervous system: what, when, and how
Abstract
p21-activated kinases (PAKs) are a family of cell division control protein 42/ras-related C3 botulinum toxin substrate 1 (Cdc42/Rac1)-activated serine/threonine kinases. Group I PAKs (PAK1-3) have distinct activation mechanisms from group II PAKs (PAK4-6) and are the focus of this review. In transformed cancer cells, PAKs regulate a variety of cellular processes and molecular pathways which are also important for myelin formation and repair in the central nervous system (CNS). De novo mutations in group I PAKs are frequently seen in children with neurodevelopmental defects and white matter anomalies. Group I PAKs regulate virtually every aspect of neuronal development and function. Yet their functions in CNS myelination and remyelination remain incompletely defined. Herein, we highlight the current understanding of PAKs in regulating cellular and molecular pathways and discuss the status of PAK-regulated pathways in oligodendrocyte development. We point out outstanding questions and future directions in the research field of group I PAKs and oligodendrocyte development.
Keywords: demyelination; differentiation; multiple sclerosis (MS); myelination; oligodendrocyte progenitor cells (OPCs); oligodendrocytes (OLs); p21-activated kinases (PAKs); remyelination.
© 2021 Cambridge Philosophical Society.
Figures
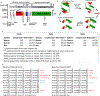
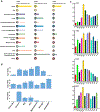
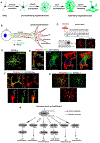
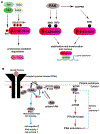
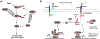
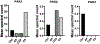
Similar articles
-
Signaling, Regulation, and Specificity of the Type II p21-activated Kinases.J Biol Chem. 2015 May 22;290(21):12975-83. doi: 10.1074/jbc.R115.650416. Epub 2015 Apr 8. J Biol Chem. 2015. PMID: 25855792 Free PMC article. Review.
-
P21 activated kinase signaling in cancer.Semin Cancer Biol. 2019 Feb;54:40-49. doi: 10.1016/j.semcancer.2018.01.006. Epub 2018 Jan 9. Semin Cancer Biol. 2019. PMID: 29330094 Review.
-
Group I p21-activated kinases regulate thyroid cancer cell migration and are overexpressed and activated in thyroid cancer invasion.Endocr Relat Cancer. 2010 Oct 29;17(4):989-99. doi: 10.1677/ERC-10-0168. Print 2010 Dec. Endocr Relat Cancer. 2010. PMID: 20817787 Free PMC article.
-
PAK1 Positively Regulates Oligodendrocyte Morphology and Myelination.J Neurosci. 2021 Mar 3;41(9):1864-1877. doi: 10.1523/JNEUROSCI.0229-20.2021. Epub 2021 Jan 21. J Neurosci. 2021. PMID: 33478987 Free PMC article.
-
Group II p21-activated kinases as therapeutic targets in gastrointestinal cancer.World J Gastroenterol. 2016 Jan 21;22(3):1224-35. doi: 10.3748/wjg.v22.i3.1224. World J Gastroenterol. 2016. PMID: 26811660 Free PMC article. Review.
Cited by
-
Control of OPC proliferation and repopulation by the intellectual disability gene PAK1 under homeostatic and demyelinating conditions.bioRxiv [Preprint]. 2024 Apr 29:2024.04.26.591153. doi: 10.1101/2024.04.26.591153. bioRxiv. 2024. PMID: 38746444 Free PMC article. Preprint.
-
Targeting RAC1 reactivates pyroptosis to reverse paclitaxel resistance in ovarian cancer by suppressing P21-activated kinase 4.MedComm (2020). 2024 Sep 2;5(9):e719. doi: 10.1002/mco2.719. eCollection 2024 Sep. MedComm (2020). 2024. PMID: 39224538 Free PMC article.
-
A de novo variant in PAK2 detected in an individual with Knobloch type 2 syndrome.bioRxiv [Preprint]. 2024 Apr 22:2024.04.18.590108. doi: 10.1101/2024.04.18.590108. bioRxiv. 2024. PMID: 38712026 Free PMC article. Preprint.
-
The molecular basis of p21-activated kinase-associated neurodevelopmental disorders: From genotype to phenotype.Front Neurosci. 2023 Mar 2;17:1123784. doi: 10.3389/fnins.2023.1123784. eCollection 2023. Front Neurosci. 2023. PMID: 36937657 Free PMC article. Review.
References
-
- Allen KM, Gleeson JG, Bagrodia S, Partington MW, MacMillan JC, Cerione RA, Mulley JC & Walsh CA (1998). PAK3 mutation in nonsyndromic X-linked mental retardation. Nature Genetics 20(1), 25–30. - PubMed
-
- Asrar S, Meng Y, Zhou Z, Todorovski Z, Huang WW & Jia Z (2009). Regulation of hippocampal long-term potentiation by p21-activated protein kinase 1 (PAK1). Neuropharmacology 56(1), 73–80. - PubMed
-
- Banerjee M, Worth D, Prowse DM & Nikolic M (2002). Pak1 phosphorylation on t212 affects microtubules in cells undergoing mitosis. Current Biology 12(14), 1233–1239. - PubMed
Publication types
MeSH terms
Substances
Grants and funding
LinkOut - more resources
Full Text Sources
Research Materials
Miscellaneous