Ochratoxin A Induces Steatosis via PPARγ-CD36 Axis
- PMID: 34822586
- PMCID: PMC8620754
- DOI: 10.3390/toxins13110802
Ochratoxin A Induces Steatosis via PPARγ-CD36 Axis
Abstract
Ochratoxin A(OTA) is considered to be one of the most important contaminants of food and feed worldwide. The liver is one of key target organs for OTA to exert its toxic effects. Due to current lifestyle and diet, nonalcoholic fatty liver disease (NAFLD) has been the most common liver disease. To examine the potential effect of OTA on hepatic lipid metabolism and NAFLD, C57BL/6 male mice received 1 mg/kg OTA by gavage daily. Compared with controls, OTA increased lipid deposition and TG accumulation in mouse livers. In vitro OTA treatment also promoted lipid droplets accumulation in primary hepatocytes and HepG2 cells. Mechanistically, OTA prevented PPARγ degradation by reducing the interaction between PPARγ and its E3 ligase SIAH2, which led to activation of PPARγ signaling pathway. Furthermore, downregulation or inhibition of CD36, a known of PPARγ, alleviated OTA-induced lipid droplets deposition and TG accumulation. Therefore, OTA induces hepatic steatosis via PPARγ-CD36 axis, suggesting that OTA has an impact on liver lipid metabolism and may contribute to the development of metabolic diseases.
Keywords: OTA; PPAR; fatty liver disease; lipid metabolism.
Conflict of interest statement
None of the authors participated in this study have anything to disclose regarding conflict of interest.
Figures
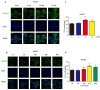
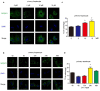
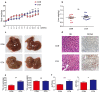
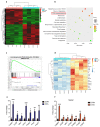
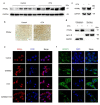
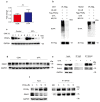
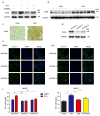
Similar articles
-
Epigenetic Regulation of Peroxisome Proliferator-Activated Receptor Gamma Mediates High-Fat Diet-Induced Non-Alcoholic Fatty Liver Disease.Cells. 2021 May 31;10(6):1355. doi: 10.3390/cells10061355. Cells. 2021. PMID: 34072832 Free PMC article.
-
Osteoprotegerin Promotes Liver Steatosis by Targeting the ERK-PPAR-γ-CD36 Pathway.Diabetes. 2019 Oct;68(10):1902-1914. doi: 10.2337/db18-1055. Epub 2019 Jul 10. Diabetes. 2019. PMID: 31292134
-
Secretory Galectin-3 promotes hepatic steatosis via regulation of the PPARγ/CD36 signaling pathway.Cell Signal. 2021 Aug;84:110043. doi: 10.1016/j.cellsig.2021.110043. Epub 2021 May 13. Cell Signal. 2021. PMID: 33991615
-
PEGylated Curcumin Derivative Attenuates Hepatic Steatosis via CREB/PPAR-γ/CD36 Pathway.Biomed Res Int. 2017;2017:8234507. doi: 10.1155/2017/8234507. Epub 2017 Jul 9. Biomed Res Int. 2017. PMID: 28770225 Free PMC article.
-
Ochratoxin A-The Current Knowledge Concerning Hepatotoxicity, Mode of Action and Possible Prevention.Molecules. 2023 Sep 14;28(18):6617. doi: 10.3390/molecules28186617. Molecules. 2023. PMID: 37764392 Free PMC article. Review.
Cited by
-
Lack of TRPV1 Channel Modulates Mouse Gene Expression and Liver Proteome with Glucose Metabolism Changes.Int J Mol Sci. 2022 Jun 24;23(13):7014. doi: 10.3390/ijms23137014. Int J Mol Sci. 2022. PMID: 35806020 Free PMC article.
-
Puerarin Prevents Bisphenol S Induced Lipid Accumulation by Reducing Liver Lipid Synthesis and Promoting Lipid Metabolism in C57BL/6J Mice.Toxics. 2023 Aug 26;11(9):736. doi: 10.3390/toxics11090736. Toxics. 2023. PMID: 37755746 Free PMC article.
-
Ochratoxin A: Overview of Prevention, Removal, and Detoxification Methods.Toxins (Basel). 2023 Sep 8;15(9):565. doi: 10.3390/toxins15090565. Toxins (Basel). 2023. PMID: 37755991 Free PMC article. Review.
-
Ochratoxin A induces abnormal tryptophan metabolism in the intestine and liver to activate AMPK signaling pathway.J Anim Sci Biotechnol. 2023 Sep 8;14(1):125. doi: 10.1186/s40104-023-00912-6. J Anim Sci Biotechnol. 2023. PMID: 37684661 Free PMC article.
-
Surfactant protein A promotes western diet-induced hepatic steatosis and fibrosis in mice.Sci Rep. 2024 Mar 29;14(1):7464. doi: 10.1038/s41598-024-58291-5. Sci Rep. 2024. PMID: 38553537 Free PMC article.
References
Publication types
MeSH terms
Substances
LinkOut - more resources
Full Text Sources
Medical
Miscellaneous