Decellularization Strategies for Regenerating Cardiac and Skeletal Muscle Tissues
- PMID: 35295645
- PMCID: PMC8918733
- DOI: 10.3389/fbioe.2022.831300
Decellularization Strategies for Regenerating Cardiac and Skeletal Muscle Tissues
Abstract
Cardiovascular disease is the leading cause of death worldwide and is associated with approximately 17.9 million deaths each year. Musculoskeletal conditions affect more than 1.71 billion people globally and are the leading cause of disability. These two areas represent a massive global health burden that is perpetuated by a lack of functionally restorative treatment options. The fields of regenerative medicine and tissue engineering offer great promise for the development of therapies to repair damaged or diseased tissues. Decellularized tissues and extracellular matrices are cornerstones of regenerative biomaterials and have been used clinically for decades and many have received FDA approval. In this review, we first discuss and compare methods used to produce decellularized tissues and ECMs from cardiac and skeletal muscle. We take a focused look at how different biophysical properties such as spatial topography, extracellular matrix composition, and mechanical characteristics influence cell behavior and function in the context of regenerative medicine. Lastly, we describe emerging research and forecast the future high impact applications of decellularized cardiac and skeletal muscle that will drive novel and effective regenerative therapies.
Keywords: ECM; cardiac engineered tissue; dECM; decellularized extracellular matrix; decellularized heart; decellularized muscle; extracellular matrix; skeletal muscle engineering.
Copyright © 2022 Tan, Helms and Nakayama.
Conflict of interest statement
The authors declare that the research was conducted in the absence of any commercial or financial relationships that could be construed as a potential conflict of interest.
Figures
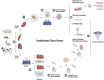
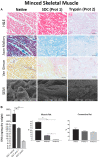
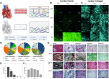
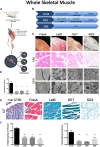
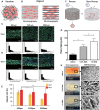
Similar articles
-
Decellularized Extracellular Matrix for Tissue Engineering (Review).Sovrem Tekhnologii Med. 2022;14(3):57-68. doi: 10.17691/stm2022.14.3.07. Epub 2022 May 28. Sovrem Tekhnologii Med. 2022. PMID: 37064810 Free PMC article. Review.
-
Decellularized skeletal muscle: A versatile biomaterial in tissue engineering and regenerative medicine.Biomaterials. 2022 Apr;283:121436. doi: 10.1016/j.biomaterials.2022.121436. Epub 2022 Feb 23. Biomaterials. 2022. PMID: 35248912 Review.
-
Preparation and Use of Decellularized Extracellular Matrix for Tissue Engineering.J Funct Biomater. 2022 Nov 14;13(4):240. doi: 10.3390/jfb13040240. J Funct Biomater. 2022. PMID: 36412881 Free PMC article. Review.
-
Recent advances in soluble decellularized extracellular matrix for heart tissue engineering and organ modeling.J Biomater Appl. 2023 Nov;38(5):577-604. doi: 10.1177/08853282231207216. J Biomater Appl. 2023. PMID: 38006224 Free PMC article. Review.
-
Decellularized extracellular matrix biomaterials for regenerative therapies: Advances, challenges and clinical prospects.Bioact Mater. 2023 Oct 4;32:98-123. doi: 10.1016/j.bioactmat.2023.09.017. eCollection 2024 Feb. Bioact Mater. 2023. PMID: 37927899 Free PMC article. Review.
Cited by
-
Three-dimensional heart extracellular matrix enhances chemically induced direct cardiac reprogramming.Sci Adv. 2022 Dec 14;8(50):eabn5768. doi: 10.1126/sciadv.abn5768. Epub 2022 Dec 14. Sci Adv. 2022. PMID: 36516259 Free PMC article.
-
Differentiating Human Pluripotent Stem Cells to Cardiomyocytes Using Purified Extracellular Matrix Proteins.Bioengineering (Basel). 2022 Nov 22;9(12):720. doi: 10.3390/bioengineering9120720. Bioengineering (Basel). 2022. PMID: 36550926 Free PMC article. Review.
-
Decellularized Extracellular Matrix Scaffolds for Cardiovascular Tissue Engineering: Current Techniques and Challenges.Int J Mol Sci. 2022 Oct 27;23(21):13040. doi: 10.3390/ijms232113040. Int J Mol Sci. 2022. PMID: 36361824 Free PMC article. Review.
-
Decellularized extracellular matrix-based composite scaffolds for tissue engineering and regenerative medicine.Regen Biomater. 2023 Dec 1;11:rbad107. doi: 10.1093/rb/rbad107. eCollection 2024. Regen Biomater. 2023. PMID: 38173774 Free PMC article. Review.
-
Recent Advances in Decellularized Extracellular Matrix-Based Bioinks for 3D Bioprinting in Tissue Engineering.Materials (Basel). 2023 Apr 18;16(8):3197. doi: 10.3390/ma16083197. Materials (Basel). 2023. PMID: 37110034 Free PMC article. Review.
References
-
- Akhyari P., Aubin H., Gwanmesia P., Barth M., Hoffmann S., Huelsmann J., et al. (2011). The Quest for an Optimized Protocol for Whole-Heart Decellularization: a Comparison of Three Popular and a Novel Decellularization Technique and Their Diverse Effects on Crucial Extracellular Matrix Qualities. Tissue Eng. C: Methods 17 (9), 915–926. 10.1089/ten.TEC.2011.0210 - DOI - PubMed
Publication types
Grants and funding
LinkOut - more resources
Full Text Sources