Improved loss-of-function CRISPR-Cas9 genome editing in human cells concomitant with inhibition of TGF-β signaling
- PMID: 35402072
- PMCID: PMC8961078
- DOI: 10.1016/j.omtn.2022.03.003
Improved loss-of-function CRISPR-Cas9 genome editing in human cells concomitant with inhibition of TGF-β signaling
Abstract
Strategies to modulate cellular DNA repair pathways hold immense potential to enhance the efficiency of CRISPR-Cas9 genome editing platform. In the absence of a repair template, CRISPR-Cas9-induced DNA double-strand breaks are repaired by the endogenous cellular DNA repair pathways to generate loss-of-function edits. Here, we describe a reporter-based assay for expeditious measurement of loss-of-function editing by CRISPR-Cas9. An unbiased chemical screen performed using this assay enabled the identification of small molecules that promote loss-of-function editing. Iterative rounds of screens reveal Repsox, a TGF-β signaling inhibitor, as a CRISPR-Cas9 editing efficiency enhancer. Repsox invariably increased CRISPR-Cas9 editing in a panel of commonly used cell lines in biomedical research and primary cells. Furthermore, Repsox-mediated editing enhancement in primary human CD4+ T cells enabled the generation of HIV-1-resistant cells with high efficiency. This study demonstrates the potential of transiently targeting cellular pathways by small molecules to improve genome editing for research applications and is expected to benefit gene therapy efforts.
Keywords: CRISPR-Cas9; Cas9 VLPs; DNA repair; Repsox; TGF-β signaling; editing in primary T cells; genome editing; small molecules.
© 2022 The Author(s).
Conflict of interest statement
The authors declare no competing interests.
Figures
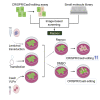
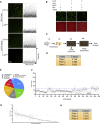
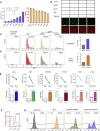
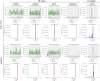
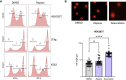
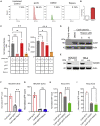
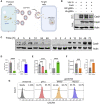
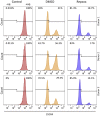
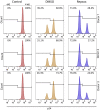
Similar articles
-
Gene Therapy with CRISPR/Cas9 Coming to Age for HIV Cure.AIDS Rev. 2017 Oct-Dec;19(3):167-172. AIDS Rev. 2017. PMID: 29019352
-
Advance trends in targeting homology-directed repair for accurate gene editing: An inclusive review of small molecules and modified CRISPR-Cas9 systems.Bioimpacts. 2022;12(4):371-391. doi: 10.34172/bi.2022.23871. Epub 2022 Jun 22. Bioimpacts. 2022. PMID: 35975201 Free PMC article. Review.
-
Single-Strand Annealing Plays a Major Role in Double-Strand DNA Break Repair following CRISPR-Cas9 Cleavage in Leishmania.mSphere. 2019 Aug 21;4(4):e00408-19. doi: 10.1128/mSphere.00408-19. mSphere. 2019. PMID: 31434745 Free PMC article.
-
Approaches to Enhance Precise CRISPR/Cas9-Mediated Genome Editing.Int J Mol Sci. 2021 Aug 9;22(16):8571. doi: 10.3390/ijms22168571. Int J Mol Sci. 2021. PMID: 34445274 Free PMC article. Review.
-
Elevated expression of exogenous RAD51 enhances the CRISPR/Cas9-mediated genome editing efficiency.BMB Rep. 2023 Feb;56(2):102-107. doi: 10.5483/BMBRep.2022-0149. BMB Rep. 2023. PMID: 36513383 Free PMC article.
Cited by
-
Single cell transcriptome analyses of the developing zebrafish eye- perspectives and applications.Front Cell Dev Biol. 2023 Jun 29;11:1213382. doi: 10.3389/fcell.2023.1213382. eCollection 2023. Front Cell Dev Biol. 2023. PMID: 37457291 Free PMC article. Review.
-
HIV-1 Vpr induces ciTRAN to prevent transcriptional repression of the provirus.Sci Adv. 2023 Sep 8;9(36):eadh9170. doi: 10.1126/sciadv.adh9170. Epub 2023 Sep 6. Sci Adv. 2023. PMID: 37672576 Free PMC article.
References
Grants and funding
LinkOut - more resources
Full Text Sources
Research Materials