Covariation of brain and skull shapes as a model to understand the role of crosstalk in development and evolution
- PMID: 36377237
- PMCID: PMC9839637
- DOI: 10.1111/ede.12421
Covariation of brain and skull shapes as a model to understand the role of crosstalk in development and evolution
Abstract
Covariation among discrete phenotypes can arise due to selection for shared functions, and/or shared genetic and developmental underpinnings. The consequences of such phenotypic integration are far-reaching and can act to either facilitate or limit morphological variation. The vertebrate brain is known to act as an "organizer" of craniofacial development, secreting morphogens that can affect the shape of the growing neurocranium, consistent with roles for pleiotropy in brain-neurocranium covariation. Here, we test this hypothesis in cichlid fishes by first examining the degree of shape integration between the brain and the neurocranium using three-dimensional geometric morphometrics in an F5 hybrid population, and then genetically mapping trait covariation using quantitative trait loci (QTL) analysis. We observe shape associations between the brain and the neurocranium, a pattern that holds even when we assess associations between the brain and constituent parts of the neurocranium: the rostrum and braincase. We also recover robust genetic signals for both hard- and soft-tissue traits and identify a genomic region where QTL for the brain and braincase overlap, implicating a role for pleiotropy in patterning trait covariation. Fine mapping of the overlapping genomic region identifies a candidate gene, notch1a, which is known to be involved in patterning skeletal and neural tissues during development. Taken together, these data offer a genetic hypothesis for brain-neurocranium covariation, as well as a potential mechanism by which behavioral shifts may simultaneously drive rapid change in neuroanatomy and craniofacial morphology.
Keywords: bone; brain; development; geometric morphometrics; phenotypic integration.
© 2022 The Authors. Evolution & Development published by Wiley Periodicals LLC.
Figures
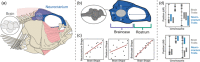
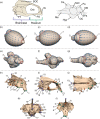
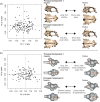
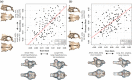
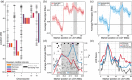
Similar articles
-
Weak genetic signal for phenotypic integration implicates developmental processes as major regulators of trait covariation.Mol Ecol. 2021 Jan;30(2):464-480. doi: 10.1111/mec.15748. Epub 2020 Dec 6. Mol Ecol. 2021. PMID: 33231336 Free PMC article.
-
How to tuna fish: constraint, convergence, and integration in the neurocranium of pelagiarian fishes.Evolution. 2023 Jun 1;77(6):1277-1288. doi: 10.1093/evolut/qpad056. Evolution. 2023. PMID: 36995728
-
Why the short face? Developmental disintegration of the neurocranium drives convergent evolution in neotropical electric fishes.Ecol Evol. 2017 Feb 15;7(6):1783-1801. doi: 10.1002/ece3.2704. eCollection 2017 Mar. Ecol Evol. 2017. PMID: 28331588 Free PMC article.
-
Patterns of integration and modularity in the primate skeleton: a review.J Anthropol Sci. 2022 Dec 30;100:109-140. doi: 10.4436/JASS.10012. J Anthropol Sci. 2022. PMID: 36565456 Review.
-
The neural crest and origin of the neurocranium in vertebrates.Genesis. 2018 Jun;56(6-7):e23213. doi: 10.1002/dvg.23213. Genesis. 2018. PMID: 30134067 Review.
Cited by
-
A brain-wide analysis maps structural evolution to distinct anatomical module.Elife. 2023 Jul 27;12:e80777. doi: 10.7554/eLife.80777. Elife. 2023. PMID: 37498318 Free PMC article.
-
Dynamic evolutionary interplay between ontogenetic skull patterning and whole-head integration.Nat Ecol Evol. 2024 Mar;8(3):536-551. doi: 10.1038/s41559-023-02295-3. Epub 2024 Jan 10. Nat Ecol Evol. 2024. PMID: 38200368
References
-
- Adams, D. C. (2016). Evaluating modularity in morphometric data: Challenges with the RV coefficient and a new test measure. Methods in Ecology and Evolution, 7, 565–572.
-
- Adams, D. C. , & Collyer, M. L. (2016). On the comparison of the strength of morphological integration across morphometric datasets. Evolution, 70, 2623–2631. - PubMed
-
- Adams, D. C. , Collyer, M. L. , Kaliontzopoulou, A. , & Baken, E. K. (2021). Geomorph: Software for geometric morphometric analyses. R package version 4.0.4. https://cran.r-project.org/package=geomorph
-
- Albertson, R. C. (2008). Morphological divergence predicts habitat partitioning in a Lake Malawi Cichlid species complex. Copeia, 2008, 689–698.
Publication types
MeSH terms
Grants and funding
LinkOut - more resources
Full Text Sources
Miscellaneous