Accurate detection of identity-by-descent segments in human ancient DNA
- PMID: 38123640
- PMCID: PMC10786714
- DOI: 10.1038/s41588-023-01582-w
Accurate detection of identity-by-descent segments in human ancient DNA
Abstract
Long DNA segments shared between two individuals, known as identity-by-descent (IBD), reveal recent genealogical connections. Here we introduce ancIBD, a method for identifying IBD segments in ancient human DNA (aDNA) using a hidden Markov model and imputed genotype probabilities. We demonstrate that ancIBD accurately identifies IBD segments >8 cM for aDNA data with an average depth of >0.25× for whole-genome sequencing or >1× for 1240k single nucleotide polymorphism capture data. Applying ancIBD to 4,248 ancient Eurasian individuals, we identify relatives up to the sixth degree and genealogical connections between archaeological groups. Notably, we reveal long IBD sharing between Corded Ware and Yamnaya groups, indicating that the Yamnaya herders of the Pontic-Caspian Steppe and the Steppe-related ancestry in various European Corded Ware groups share substantial co-ancestry within only a few hundred years. These results show that detecting IBD segments can generate powerful insights into the growing aDNA record, both on a small scale relevant to life stories and on a large scale relevant to major cultural-historical events.
© 2023. The Author(s).
Conflict of interest statement
The authors declare no competing interests.
Figures
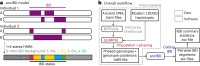
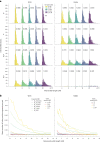
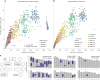
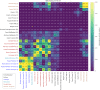
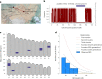
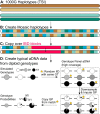
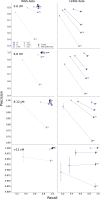
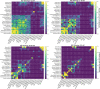
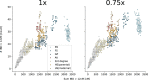
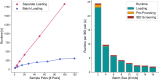
Update of
-
ancIBD - Screening for identity by descent segments in human ancient DNA.bioRxiv [Preprint]. 2023 Mar 9:2023.03.08.531671. doi: 10.1101/2023.03.08.531671. bioRxiv. 2023. Update in: Nat Genet. 2024 Jan;56(1):143-151. doi: 10.1038/s41588-023-01582-w. PMID: 36945531 Free PMC article. Updated. Preprint.
Similar articles
-
ancIBD - Screening for identity by descent segments in human ancient DNA.bioRxiv [Preprint]. 2023 Mar 9:2023.03.08.531671. doi: 10.1101/2023.03.08.531671. bioRxiv. 2023. Update in: Nat Genet. 2024 Jan;56(1):143-151. doi: 10.1038/s41588-023-01582-w. PMID: 36945531 Free PMC article. Updated. Preprint.
-
Mitochondrial genomes reveal an east to west cline of steppe ancestry in Corded Ware populations.Sci Rep. 2018 Aug 2;8(1):11603. doi: 10.1038/s41598-018-29914-5. Sci Rep. 2018. PMID: 30072694 Free PMC article.
-
The genetic and cultural impact of the Steppe migration into Europe.Ann Hum Biol. 2021 May;48(3):223-233. doi: 10.1080/03014460.2021.1942984. Ann Hum Biol. 2021. PMID: 34459341
-
Detection of identity by descent using next-generation whole genome sequencing data.BMC Bioinformatics. 2012 Jun 6;13:121. doi: 10.1186/1471-2105-13-121. BMC Bioinformatics. 2012. PMID: 22672699 Free PMC article.
-
Toward high-resolution population genomics using archaeological samples.DNA Res. 2016 Aug;23(4):295-310. doi: 10.1093/dnares/dsw029. Epub 2016 Jul 19. DNA Res. 2016. PMID: 27436340 Free PMC article. Review.
Cited by
-
Ancient Rapanui genomes reveal resilience and pre-European contact with the Americas.Nature. 2024 Sep;633(8029):389-397. doi: 10.1038/s41586-024-07881-4. Epub 2024 Sep 11. Nature. 2024. PMID: 39261618 Free PMC article.
-
Shared chromosomal segments connect ancient human societies.Nat Genet. 2024 Jan;56(1):10-11. doi: 10.1038/s41588-023-01606-5. Nat Genet. 2024. PMID: 38123641 No abstract available.
-
The rise and transformation of Bronze Age pastoralists in the Caucasus.Nature. 2024 Nov;635(8040):917-925. doi: 10.1038/s41586-024-08113-5. Epub 2024 Oct 30. Nature. 2024. PMID: 39478221 Free PMC article.
-
Long shared haplotypes identify the Southern Urals as a primary source for the 10th century Hungarians.bioRxiv [Preprint]. 2024 Jul 23:2024.07.21.599526. doi: 10.1101/2024.07.21.599526. bioRxiv. 2024. PMID: 39091721 Free PMC article. Preprint.
-
Review: Computational analysis of human skeletal remains in ancient DNA and forensic genetics.iScience. 2023 Oct 4;26(11):108066. doi: 10.1016/j.isci.2023.108066. eCollection 2023 Nov 17. iScience. 2023. PMID: 37927550 Free PMC article. Review.
References
MeSH terms
Substances
Grants and funding
LinkOut - more resources
Full Text Sources