Laboratory realization of relativistic pair-plasma beams
- PMID: 38866733
- PMCID: PMC11169600
- DOI: 10.1038/s41467-024-49346-2
Laboratory realization of relativistic pair-plasma beams
Abstract
Relativistic electron-positron plasmas are ubiquitous in extreme astrophysical environments such as black-hole and neutron-star magnetospheres, where accretion-powered jets and pulsar winds are expected to be enriched with electron-positron pairs. Their role in the dynamics of such environments is in many cases believed to be fundamental, but their behavior differs significantly from typical electron-ion plasmas due to the matter-antimatter symmetry of the charged components. So far, our experimental inability to produce large yields of positrons in quasi-neutral beams has restricted the understanding of electron-positron pair plasmas to simple numerical and analytical studies, which are rather limited. We present the first experimental results confirming the generation of high-density, quasi-neutral, relativistic electron-positron pair beams using the 440 GeV/c beam at CERN's Super Proton Synchrotron (SPS) accelerator. Monte Carlo simulations agree well with the experimental data and show that the characteristic scales necessary for collective plasma behavior, such as the Debye length and the collisionless skin depth, are exceeded by the measured size of the produced pair beams. Our work opens up the possibility of directly probing the microphysics of pair plasmas beyond quasi-linear evolution into regimes that are challenging to simulate or measure via astronomical observations.
© 2024. The Author(s).
Conflict of interest statement
The authors declare no competing interests.
Figures
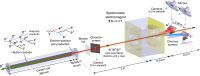
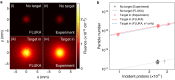
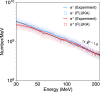
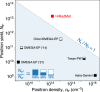
Similar articles
-
Generation of neutral and high-density electron-positron pair plasmas in the laboratory.Nat Commun. 2015 Apr 23;6:6747. doi: 10.1038/ncomms7747. Nat Commun. 2015. PMID: 25903920 Free PMC article.
-
Characterization of relativistic electron-positron beams produced with laser-accelerated GeV electrons.Sci Rep. 2023 Jan 6;13(1):310. doi: 10.1038/s41598-023-27617-0. Sci Rep. 2023. PMID: 36609530 Free PMC article.
-
Experimental Observation of a Current-Driven Instability in a Neutral Electron-Positron Beam.Phys Rev Lett. 2017 Nov 3;119(18):185002. doi: 10.1103/PhysRevLett.119.185002. Epub 2017 Nov 3. Phys Rev Lett. 2017. PMID: 29219555
-
PIC methods in astrophysics: simulations of relativistic jets and kinetic physics in astrophysical systems.Living Rev Comput Astrophys. 2021;7(1):1. doi: 10.1007/s41115-021-00012-0. Epub 2021 Jul 8. Living Rev Comput Astrophys. 2021. PMID: 34722863 Free PMC article. Review.
-
Neutrino transport in general relativistic neutron star merger simulations.Living Rev Comput Astrophys. 2023;9(1):1. doi: 10.1007/s41115-023-00016-y. Epub 2023 Feb 23. Living Rev Comput Astrophys. 2023. PMID: 36852009 Free PMC article. Review.
References
-
- Bambi, C. (ed.) Astrophysics of black holes: from fundamental aspects to latest developments (Springer, Berlin/Heidelberg, 2016).
-
- Arons J. Some problems of pulsar physics or I’m madly in love with electricity. Space Sci. Rev. 1979;24:437–510. doi: 10.1007/BF00172212. - DOI
-
- Breit G, Wheeler JA. Collision of two light quanta. Phys. Rev. 1934;46:1087. doi: 10.1103/PhysRev.46.1087. - DOI
-
- Schwinger J. On gauge invariance and vacuum polarization. Phys. Rev. 1951;82:664. doi: 10.1103/PhysRev.82.664. - DOI
-
- Erber T. High-energy electromagnetic conversion processes in intense magnetic fields. Rev. Mod. Phys. 1966;38:626. doi: 10.1103/RevModPhys.38.626. - DOI
LinkOut - more resources
Full Text Sources